1 Introduction
1.1 A Heat Transfer Fluid
Man has used the technique of converting liquid water into steam and using the expansive force of this vapour as far back as 150 B.C. [1]. Despite this, it took over 1800 years for humans to harness the practical features of steam to conduct productive work. Around the year 1711, the first commercial piston-operated mine pump was invented. However, it wasn’t until the latter half of the nineteenth century that the first steam-operated central power station was introduced. In 1882, the Holborn Viaduct power station in London and Pearl Street Station in New York were put into service using reciprocating steam engines.
Steam provides a mechanism for transporting controllable amounts of energy from a central boiler, where it is efficiently and economically generated, to the point of use. Steam is one of the most popular means of conveying heat energy for many reasons. Its use is widespread throughout the industry for a wide-ranging spectrum of tasks, from space heating to mechanical power production and process applications. This is why some consider steam to be the ultimate energy fluid [2].
The capability of steam to hold a large amount of energy on a per weight basis (1000–1200Btu/lb) makes it ideal for use as an energy transport medium. Given that most of the steam heat energy is in the form of latent heat, significant amounts of energy can be transferred efficiently at a constant temperature, which is helpful for many process heating applications. Steam has come a long way from its conventional ties with locomotives and the Industrial Revolution in the nineteenth century. Steam is now a necessary and important aspect of modern technology. Our chemical, medical, food, beverage, textile, power, heating, and transportation industries would be paralysed without the use of steam.

Figure 1. Steam going out from cooling towers at Doel nuclear power station in Belgium. Source: https://commons.wikimedia.org/wiki/File:Kerncentrale_Doel_in_werking.jpg
1.2 What is Steam?
Water can exist in the form of a liquid (water), a gas (steam), or a solid (ice). This article will concentrate on the fluid phases and the equipment required to facilitate the change from one to the other. When heat energy is supplied to liquid water, its temperature ascends until a value is reached at which the water can not any more exist as a liquid. We call this tipping point the “saturation” point. As more energy is added, some of the water will boil and become steam. This evaporation process requires relatively large amounts of power, and while it is being added, the liquid water and the vapour are both at the same temperature.
Steam creates pressure when it forms in a closed vessel, allowing it to move anywhere to a lower pressure, such as through piping and distant equipment. Allowing the steam to cool will also release the energy that was used to evaporate it. These boiling, transfer and condensing events provide a simple mechanism to transfer power from one place to another, hence the basis of a steam system. Interestingly, steam is colourless; the white colour often seen when steam discharges into the atmosphere corresponds to condensed water vapour in the steam.
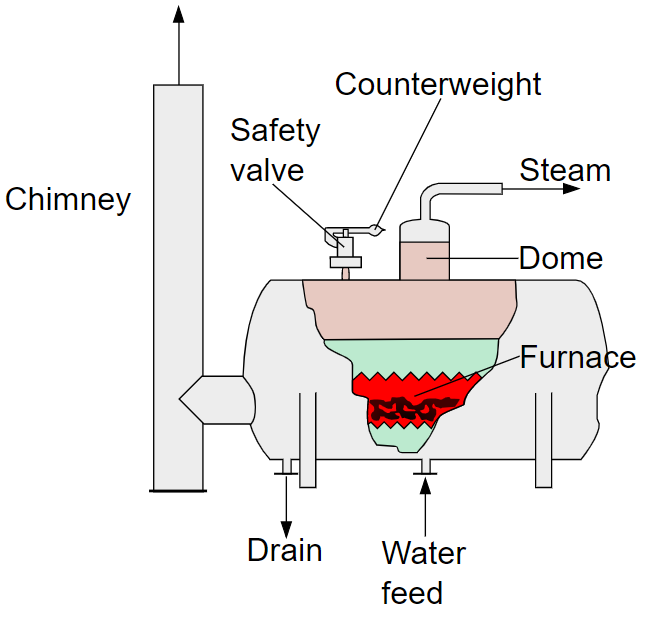
Figure 2. Diagram of a steam boiler. Source: https://commons.wikimedia.org/wiki/File:Steam_Boiler_1_english.svg
2 Steam Generation
2.1 Boiling and Circulation
The boiling of a liquid refers to a condition where vapour bubbles form on the heating surface of the liquid. Bubble formation is influenced by fluid characteristics as well as operational and surface conditions. When heat is applied to a liquid, it does not raise its temperature over the saturation temperature corresponding to its pressure; instead, the energy employed causes a phase shift from a liquid to a gaseous state, such as from water to steam. Boiling is a process that takes place at a constant pressure and temperature.
The temperature at which a liquid’s vapour pressure equals the gas pressure above it is known as the boiling point. Saturation temperature is another name for this temperature. The boiling point of a liquid in an open vessel is the temperature at which its vapour pressure equals the external pressure at one atmosphere. The boiling point of a liquid is reduced when the external pressure is less than one atmosphere. The boiling point of a liquid begins to climb when the external pressure rises above one atmosphere.The boiling point of a liquid is reduced when the external pressure is less than one atmosphere. The boiling point of a liquid begins to climb when the external pressure rises above one atmosphere.
Boiling happens when heat is applied to a liquid at a rate that causes it to reach its saturation temperature, which corresponds to the total pressure over the liquid’s free surface. When the vessel is open to the atmosphere, the vapour completely displaces the air from the liquid’s surface, however when the vessel is closed to the atmosphere, the vapour is evacuated through diffusion. The heat of evaporation or heat of vaporisation is the heat that converts water to steam.
The heat transfer that takes place from the wall to the liquid during boiling is given by the convective heat transfer equation:
where Q: Rate of heat flow (W); h: Heat transfer coefficient (W/m2); A: Area across which heat flow takes place (m2); ΔT: Difference in temperature between the heating surface and the temperature of the fluid (K).
The “heat flux” at the heating surface is found from the previous eq. as:
In drum-type subcritical boilers, water flows from the drum through downcomers to the bottom of the furnace, then moves up through risers or evaporator tubes and returns to the drum. It can be seen that the water in the downcomers does not receive any heat, while risers, which form furnace walls, absorb the heat of combustion of the fuel. As a result, the water inside the riser tubes is heated, and gradually, steam bubbles form in the water.
This mixture of water and steam in the riser tubes has a much lower density than the density of water alone in the downcomers. A density difference thus generated results in a static head difference causing the thermo-siphon effect, which provides the driving force for a downward flow in downcomers and an upward flow in risers. Since the fluid flow is generated by density difference alone, this is known as natural circulation in boilers [3].
To maintain a continuous fluid circulation, the fluid flow must overcome friction losses in downcomer and riser tubes, headers, bends, etc. One way of overcoming resistance due to friction is by increasing heat input to the furnace. As the heat absorption increases, the density difference between the fluid in the downcomers and the fluid in the risers also increases, resulting in an increase in fluid flow.
However, an increase in fluid flow, in turn, also causes an increase in friction loss. As a result, a point is reached when the rate of growth in friction loss surpasses the benefit of the enhanced density difference, where the rate of fluid flow starts to “drop.” Hence, all natural circulation boilers are designed to operate below the point of “drop.” The phenomenon of rising flow-rising heat input tends to make a natural circulation boiler self-compensating in the case of sudden overloads, changes in heat-absorbing surface conditions, non-uniform burner disposition, etc.
The process of circulation, be it natural or forced, is dominated by what is called the circulation ratio, which is defined as the ratio of the “quantity of water in circulation” to the “quantity of steam produced.”
2.2 Design
Combustion converts the chemical energy in the fuel to heat energy, which is transferred to low-temperature water in the steam generator, where it is converted to high-energy steam for use in the steam turbine.
The salient features of this heat-transfer process encompass the following circuits, systems, or areas:
- Water and Steam Circuit
- Furnace
- Fuel-burning System
- Draft System
- Heat Recovery System
Water and steam circuit
The temperature of the feedwater before it enters the steam generator is lower than the saturation temperature, indicating that the feedwater is subcooled. The feedwater temperature in the steam generator is raised to near-saturation temperature in the economiser. The sensible heat for bringing the feedwater to saturation and the latent heat for evaporating saturated feedwater is added to the water wall to generate steam.
This steam is separated from the steam-water mixture coming out from the water wall and purified in the boiler drum to ensure a saturated steam supply. The saturated vapour is further superheated to attain the desired energy level.

Figure 3. Water and steam circuit. Source: doi.org/10.1016/B978-0-12-801575-9.00002-0
Furnace
In the furnace of a steam generator, fuel combustion takes place with atmospheric air to release heat. To capture the greatest heat potential of the fuel under combustion, it is critical to achieve complete combustion of the fuel under all operating conditions
System for Burning Fuel
The basic goal of a fuel-burning system is to convert the chemical energy of a fossil fuel into thermal energy in a controlled and efficient manner. The heat energy created is delivered to the steam generator’s heat-adsorbing surfaces. The burner is the most important piece of equipment for burning fossil fuels in a furnace.
An ideal fuel-burning system in a furnace is characterised by the following:
- There is no extra oxygen or unburned fuel in the combustion product.
- A low rate of auxiliary ignition energy input is required to commence and maintain the combustion reaction’s continuance.
- Wide and stable firing range
- Response time was quick in response to a request for a modification in firing rate.
- Flue-gas weight and temperature distribution in relation to parallel circuits of heat-absorbing surface
- High degree of equipment availability with a low maintenance requirement
- Safety must be the main requirement under all operating conditions
Draft system
To maintain a healthy combustion process in a furnace, it must receive a constant flow of air while also removing combustion products from the furnace without interruption. A natural draft system is one in which combustion products are released solely through a chimney.
When the chimney is augmented with forced draft (FD) fans or induced draft (ID) fans, the system is called a mechanical draft system [4]. Small steam generators employ natural draft, while large steam generators require mechanical draft to move large volumes of air and gas against flow resistance.
A furnace utilising only an FD fan is subjected to a “positive draft” (pressurised), while one using an ID fan only is subjected to a “negative draft” (subatmospheric pressure). Normally large coal-fired steam generators utilise balanced draft, employing both FD fans and ID fans.
Heat recovery system
The flue gas or combustion products leaving the convection heat-absorbing surfaces in large steam generators still carry substantial heat energy at a temperature much above the steam saturation temperature. If exhausted to the atmosphere without further heat transfer, this heat will result in significant losses. As a natural consequence of the economic operation of a steam generator, a major part of this heat is recovered by placing the economiser and, after that, the air heater in the exit flue-gas path.
Theoretically, reducing the flue-gas temperature to that of the incoming air to the air heater is possible. However, as described below, such a reduction in exit flue-gas temperature is prohibited for technical and economic considerations.
- All fossil fuels are sulphur-bearing fuels. As such, if the temperature of the output flue-gas from the air heater drops below the acid dew point of the gas-fired fuel, sulphurous or sulfuric acid will form, causing back-end corrosion in the air heater. As a consequence, both maintenance costs and plant outages will increase.
- Capital costs incurred for installing some extra heat recovery surfaces may offset the savings obtained by lowering flue-gas temperature.
In an economiser, Before entering the evaporation circuit, the incoming feedwater absorbs heat from the departing flue gases, elevating the temperature of the feedwater as close as possible but below its saturation point.
The remaining heat in flue gas downstream of the economiser is recovered in the air heater to raise the temperature of combustion air prior its entrance into the furnace. The energy thus retrieved returns to the furnace and diminishes the amount of heat that must be released by the fuel to keep the desired furnace temperature, thereby ensuring a reduction in the amount of fuel to be burned [5]. The hot air is also used to dry coal in a pulverised coal-fired boiler.
3 Steam Applications
We have discussed the usefulness of steam as a heat transfer medium and described the subsystems in detail in previous sections. Collectively, that information can be beneficial if it can all be applied to make a complete steam system.
This section will review the specific type of steam systems found in the process industry and some design considerations required for each type. Some specific applications are shown in Table 1 [6]. It is relatively easy to see that process steam systems are used to make products we use every day. Although there are dozens of steam system configurations in use today, generally, steam system configurations can be described in one of these four types of applications:
- High‐pressure steam with high condensate returns
- Low‐pressure steam with high condensate returns
- High‐ or low‐pressure steam with little or no condensate returns
- Very high‐pressure or superheated steam with some condensate returns
Each steam application requires a unique set of design criteria for optimal performance. It is not uncommon to see a combination of applications from different types employed by a single steam system (i.e., high‐pressure process steam used with low‐pressure steam heating coils). In addition to the four applications, considerations for using multiple boilers of any pressure rating and condensate return rate are described. Lastly, specialised steam using equipment like back pressure turbines (BPT), hydro heaters, superheaters, and steam mufflers are described, and guidance for their installation is presented.

Table 1. Steam System Applications. Data from the Advanced Manufacturing Office, US Department of Energy.
3.1 High‐Pressure Steam with High Condensate Returns
This type of steam application is considered a “closed” system and is the most widely used steam in the process industry (Figure 4). High‐pressure steam is usually steam at or under 300 psig. Steam in this application can be used for product heating via a shell and tube heat exchanger, steam coil in a tank, plate a frame heat exchanger, jacketed kettle, steam drum/roller, evaporator reboiler, steam jacket, steam press, or steam ironer. The steam condenses in the equipment, and the condensate is removed, recovered, and returned to the boiler room. Applications will have
the following characteristics:
- Nearly 100% condensate return
- Minimal freshwater makeup
- Minimal boiler blowdown volume
- Require relatively high steam quality
- Require minimal water treatment

Figure 4. High‐pressure closed steam system. Source: https://industrialsteam.com/high-pressure-condensate-return-hpr/
These high‐pressure high‐condensate process applications require good steam trap sizing and conservative pipe sizing; otherwise, waterlogging can occur in the steam using the equipment. Once the system is set up to operate properly, they usually are quite easy to maintain because the steam and condensate flow are relatively steady.
3.2 Low‐Pressure Steam with High Condensate Returns
Low‐pressure steam is generated and sent throughout a building or series of buildings where the steam is used for heating. These types of systems are used widely in steam heating, steam to hot water generation, or heat tracing applications (Figure 5). Typically the steam used is less than 50 psig, with 12–14 psig found most often. Schools, colleges, and older manufacturing facilities have used low-pressure steam heating systems for many years. These types of systems share the same characteristics as high‐pressure systems with high condensate returns, and only the steam quality can be much lower. It is not uncommon to see a high‐pressure process steam system used with pressure‐reducing valves (PRV) to lower steam pressure for space heating in the same facility.
Many low‐pressure steam heating systems are being replaced with high efficient hot water systems. When a process steam application calls for a process temperature less than 230°F, low‐pressure steam can be a suitable heat transfer method. Because of the high returns, water chemistry is relatively easy to maintain. In some areas of the United States, low‐pressure steam systems do not require a stationary operator, which is not the case for some high‐pressure steam systems.

Figure 5. Low‐pressure steam systems with high returns. Source: https://doi.org/10.1002/9781119085454.ch12
One caution to keep in mind for these low‐pressure steam systems is condensate lift limitations. If condensate must be pumped at any significant distance or height, the low‐pressure steam may not have enough motive force to push the condensate. If the steam pressure at a steam trap is only 10 psig, then a rise of more than 10–15 ft is enough to create waterlogging. In these applications, you should employ a Hartford loop system or drain the condensate to an atmospheric tank and electrically pump the condensate back to the boiler room.
Low‐pressure steam systems with high returns can also employ a Hartford loop design. This essentially is a closed loop system in that gravity drains the condensate back to the boiler. A Hartford loop system is simple and relatively maintenance free. Many older steam heating systems use this system configuration.
3.3 High‐ or Low‐Pressure Steam with Little or No Returns
These types of steam systems are found in sterilisation, food processing, biofuels, paper manufacturing, and any other application where steam is injected directly into the product being heated, or the condensate must be discarded. Some applications, like using steam to heat plating tanks of acid or steam tunnels for laundry, do not recover the condensate because of the risk of condensate contamination.
Steam systems with little or no returns will:
- Have a very high freshwater makeup rate
- Have a high boiler blowdown rate
- Require more water treatment technology
- May require extremely high-quality steam
This application requires some distinct design considerations to protect the product being heated. For example, sterilisers that use direct injection steam to disinfect cannot tolerate wet or dirty steam. Imagine a doctor picking up a surgery tool that has been sterilised this way that has dissolved solids spots on it [7]. Consequently, any water in the steam needs to be removed. Steam filters and separators are commonly employed in these applications. Boiler sizing also becomes a bit tricky.
Since there is a high percentage of colder makeup water, more fuel energy will be used to heat the water up to the boiling point before any steam is produced. This added sensible heat must be taken into account, or the boiler can be undersized. In many applications of this type, steam is used in batch‐type applications, and the use of steam accumulators is warranted. Such is the case for autoclaves that use steam to sterilise. Initially, the autoclave vessel is cold and will have a warm‐up load that could exceed the boiler capacity.
It should be quite apparent that a steam system application with little or no condensate returns requires the boiler size calculation to consider the energy needed to heat up the cold water makeup and account for the heat loss from the blowdown water.
3.4 High‐Pressure or Superheated Steam with Condensate Returns
This type of system is found in the process industry, where the process requires relatively high temperatures. Rubber processing, refineries, chemical manufacturing, and other industries use these systems. High steam pressure may require expensive components, and superheated steam use will require careful condensate collection systems. Remember that superheated steam is saturated steam with added sensible heat energy. Therefore, superheated steam must give up sensible and latent heat to condense fully. Correct trap selection is critical.
These types of steam systems can be susceptible to steam hammer and steam erosion across steam regulating valves. Equally important is to design for thermal expansion if long pipe runs are required. Drain legs must be installed at regular intervals to support condensate draining prior to starting up. To minimise steam hammer, individual condensate runs should also be introduced into the main condensate header via a condensate diffuser.
Regardless of the condensate return volume, when a design calls for multiple boilers, some additional design features need to be considered. A boiler master control panel should be included to ensure lead-lag sequencing of the boilers occurs. In addition, system operators should strive to operate any steam boiler in its most efficient output range, which is usually 20–80% output. When multiple boilers are tasked, the boiler master control panel will help achieve this goal. When multiple boilers are connected to a common steam header, high water protection is required for each boiler. This is because migrant steam from an operating boiler will flood an idle boiler unless a check valve is installed in the boiler steam line or a means to keep equal water levels in all boilers is used.
4 Specialised Steam Equipment
There are many types of specialised steam using equipment, but we limit our discussion in this section to four major types, BPT, hydro heaters, superheaters, and steam mufflers.
4.1 Back Pressure Turbine or BPT
BPT uses high‐pressure steam to generate electricity or provide mechanical work (i.e., shaft work). Facilities that can generate high‐pressure (>200 psig) steam but use lower pressure steam can employ a BPT. Those facilities often use PRV to step down the high‐pressure steam to lower pressure. A BPT can be used in place of a PRV.
The use of a BPT is different from a condensing turbine. In a condensing steam turbine (what is typically used to generate electricity in a steam power plant), the steam condenses and is collected and fed back to the steam generator. A BPT does not condense the steam; it just extracts enthalpy by reducing its pressure. The higher the pressure reduction across the BPT, the more energy can be extracted and eventually converted to electricity or mechanical work.
One can also calculate the amount of added steam flow required to operate the BPT. First, obtain the enthalpy loss data across the BPT and determine the added steam flow at the turbine inlet to account for the loss.
4.2 Steam Hydro Heater
A second specialised piece of steam equipment is a hydro heater. A hydro heater is actually a branded name of a steam injector manufactured by the Hydro‐Thermal Corporation. Although there are other similar functioning pieces of equipment on the market today, the name hydro heater seems to best fit how to describe the function of this machine. Hydro heaters are internally modulating steam injectors that inject steam with a 90-degree fluid flow design favourable for slurries that require high shear heating.
A liquid or slurry enters the combining tube, where steam is introduced into the fluid through a nozzle. The nozzle discharges steam at very high, often sonic velocity. The rugged nature of this high-velocity discharge enables steam to instantaneously penetrate, disperse, and efficiently mix with the slurry or liquid. Temperature is measured downstream, and feedback is sent to a controller, which modulates steam flow via an actuator linked directly to the steam nozzle plug. They are used extensively in the pulping and biorefining industries to heat the process fluid. They can be used in food processing and other manufacturing facilities with steam available and require a simple means to heat a high‐flow process stream.

Figure 6. Hydro heater. © Hydro‐Thermal Corp. Source: https://www.foodprocessing-technology.com/contractors/thermal_processing/hydro-thermal/
Essentially, the flow rate of the process stream is manually controlled by moving an adjustment cone more open or more closed. The hydro heater automatically adjusts the steam flow to maintain the temperature of the process fluid; therefore, no external steam control valve is needed. The violent mixing helps ensure the process fluid is heated uniformly and can aid in breaking up particulate mixtures [8]. In corn‐based ethanol plants, hydro heaters are used to add thermal energy to corn mash slurries and aid in gaining access to the starches inside the corn fibre shell.
There are design considerations that should be used when applying a hydro heater. The steam supply will need a minimum pressure and should be of high quality. Strainers and drip legs should always be utilised in the steam line upstream of the heater. There are process flow and pressure relationships to steam flow and pressure required through the hydro heater. Sizing is critical, and consultation with the manufacturer should occur before system design. Unmatched process flow and steam flow can create excessive vibration and noise. When tuned properly, a hydro heater is quiet and operates very efficiently. Sometimes, a valve is placed in the discharge of the heater to ensure a slight back pressure is placed on the unit to help balance flows.
4.3 Steam Superheaters
Occasionally, a process heating application may require heating a product to a temperature that makes using saturated steam impractical due to the required very high steam pressure. An independent hot oil system can be used for those applications, or a steam superheater may be employed. Hot oil systems use hot oil circulated under low pressure in lieu of steam. They can be expensive.
If a steam supply exists in a facility, an alternate solution might be to add a steam superheater to add sensible energy to the existing steam supply. Superheaters can be gas-fired, electric or use waste heat to add energy to the steam supply. Superheated steam will be at saturated steam pressure but have a higher temperature.
Superheaters are utilised to elevate the temperature of steam beyond its saturation temperature [9]. Usually, the dry and saturated steam enters the superheater via correspondingly saturated steam piping from the evaporator drum exit to the inlet header in the superheater. Subsequently, the heating surface absorbs the influx from the turbine exhaust gas and then into the steam, whose temperature increases.
At the same time, the steam flowing through the heating surface, headers, and interconnecting piping loses pressure. The heating surface is designed to deliver the required steam pressure/temperature conditions at the scope of the supply terminal point or the steam consumer. In general, the pressure loss should be minimised while maintaining strong cooling of the tubes as the saturation pressure in the evaporator increases with increasing superheater pressure loss, and the steam flow then decreases.
4.4 Steam Discharge Mufflers
It may become necessary for large process steam systems to install a steam muffler on the steam dump line. If the need for steam is suddenly stopped during a process, the excess steam produced will be vented in mass from the steam header to the boiler building exterior. This situation can result in loud, high‐pitched irritating noise [10]. This is especially true for process boilers located in municipalities with high community awareness. A steam muffler can be employed to reduce the noise level to acceptable levels. One such application is shown in Figure 7. The muffler acts similar to the one found on an automobile exhaust.
The muffler system design must take into consideration the forces created by discharging a high mass of high‐pressure steam and must account for the condensate that will likely be created in the muffler itself. Construction should be stainless steel, and a qualified structural engineer should approve the mounting.

Figure 7. Schematic of a Pulsco steam muffler/silencer. Source: https://asa.scitation.org/doi/abs/10.1121/2.0000012
5 References
[1] https://www.egr.msu.edu/~lira/supp/steam/
[2] Merritt, C. (2015). Process steam systems: a practical guide for operators, maintainers, and designers. John Wiley & Sons.
[3] Sarkar, D. K. (2015). Chapter 2-Steam Generators. Thermal Power Plant, 39-89.
[4] https://www.sciencedirect.com/topics/engineering/induced-draft
[5] https://www.thermopedia.com/content/838/
[6] Steam System Applications. (2015). Process Steam Systems, 187–203. doi:10.1002/9781119085454.ch12
[7] https://www.cdc.gov/infectioncontrol/guidelines/disinfection/sterilization/steam.html
[8] https://www.hydro-thermal.com/how-it-works
[9] Hennessey, S. P. (2017). Superheaters and reheaters. Heat Recovery Steam Generator Technology, 95–113. doi:10.1016/b978-0-08-101940-5.00006-3
[10] Tohid, U., Gubitz, K., Genger, C., Accorsi, I., Kaiser, J., & Pacheco-Vega, A. (2014, October). Parametric analysis of a Pulsco vent silencer. In Proceedings of Meetings on Acoustics 168ASA (Vol. 22, No. 1, p. 040003). Acoustical Society of America.