1 Introduction to Hydraulic Fracturing
Hydraulic fracturing is a form of Well stimulation with use in the United States dating back to the late 1940s. Early methods of Well stimulation with explosive torpedoes, acids, and other experimental methods gave way as hydraulic fracturing proved commercially successful.
This method of pumping high-pressure liquids into a wellbore proved instrumental when paired with horizontal drilling, in the U.S. Shale Revolution. Further refining this technique has reduced cost and increased productivity for oil and gas by allowing producers to gather resources from previously unavailable sources.
Hydraulic fracturing is a form of Well stimulation with use in the United States dating back to the late 1940s. Early methods of Well stimulation with explosive torpedoes, acids, and other experimental methods gave way as hydraulic fracturing proved commercially successful.
This method of pumping high-pressure liquids into a wellbore proved instrumental when paired with horizontal drilling, in the U.S. Shale Revolution. Further refining this technique has reduced cost and increased productivity for oil and gas by allowing producers to gather resources from previously unavailable sources.
Hydraulic fracturing is a form of Well stimulation with use in the United States dating back to the late 1940s. Early methods of Well stimulation with explosive torpedoes, acids, and other experimental methods gave way as hydraulic fracturing proved commercially successful.
This method of pumping high-pressure liquids into a wellbore proved instrumental when paired with horizontal drilling, in the U.S. Shale Revolution. Further refining this technique has reduced cost and increased productivity for oil and gas by allowing producers to gather resources from previously unavailable sources.

Figure 1. Horizontal well and fracturing illustration
2 Conventional and Unconventional Resources
Conventional resources are reservoirs, gas fields, or other readily accessible geologic formations, which vertical wells and traditional techniques have little difficulty producing from. Until the advent of modern hydraulic fracturing techniques, inaccessible geologic formations were identified as resource-rich yet were beyond the means of contemporary technology to produce.
Unconventional resources are tight oil or gas traps, sands, coal bed seams, salt dome traps, shales, and a wide variety of other sources. These geologic formations are readily accessible with the combined use of horizontal drilling and hydraulic fracturing.
The further exploration of production methods for unconventional resources such as methane hydrates and biogenic gas will undoubtedly play a tremendous role in the future of widely available, inexpensive, and reliable energy production.
3 Hydraulic Fracturing: The Exploration and Drilling Process (Drilling)
Exploration for hydrocarbon resources is carried out by teams of geologists, geophysicists, and technical experts who use sophisticated technologies to survey and map reservoirs and unconventional resources. Once teams have located and sufficiently mapped ideal locations, equipment, crew, and drilling rigs are brought to the location for exploratory drilling.
Depending on whether the resource is conventional or unconventional, a straightforward or complex well path trajectory will be planned. Conventional resources may be adequately addressed with a vertical well, drilled directly into the reservoir to access hydrocarbons. Unconventional resources often require a horizontally drilled well path to economically produce hydrocarbons. Whether vertically or horizontally drilled, each Wellpath may benefit from stimulation techniques.
4 Vertically and Horizontally Drilled Wells
Vertical wells are the traditional, lower-cost drilling method for accessing oil and gas resources. The trade-off for lower cost is less productivity and the inability to access unconventional resources at an economical rate. Despite the drawbacks, vertical wells benefit from a multitude of technological and methodological advancements over the decades, making them more stable, accurate, and productive than ever before.

Figure 2. Unconventional resources with deviated, horizontal, and vertical wells
Horizontal wells are considerably more complex and costly (roughly 1.4 times cost) than vertical wells. Their advantages, however, have made them the most commonly drilled well in modern oil and gas production. Horizontal directional drilling started with simply sidetracking a vertical well around an obstruction. Experimentation with well trajectory deviation methods yielded specialized bottom-hole assemblies (BHAs) and other equipment essential to precise, repeatable well deviation.
The benefit of drilling a horizontal well is increased exposure to the pay zone. The horizontal formations of unconventional resources are accessed in parallel, exposing more surface area of the Well path to the surrounding resource. Pumping high-pressure fluids into the well create microfracture networks within these hydrocarbon-bearing formations. Once created, oil and natural gas flow freely into the well at a greater speed than a simple, vertical well.
5 Hydraulic Fracturing: Well Construction (Well Control)
The planning, engineering, and construction of a well are vital to hydraulic fracturing operations. Thorough engineering and planning take place long before drilling operations commence. This is in part due to the nature of Well construction and the myriad of factors influencing well integrity.
Once these aspects of Well design are accounted for, drilling begins. As the well it drilled an iterative process of drilling, adding casing, and adding cement takes place. Reaching the target area, drilling and well construction ceases to allow hydraulic fracturing. Some wells are designed to target multiple geologic structures and will begin drilling towards a second or further goal.
5.1 Well Planning
Assessing and preparing a site requires the examination of geographic and geologic features. Examining data from surveying equipment allows crews to consider well paths with characteristics of the site such as nearby infrastructure, topography, water sources, populated areas, and land ownership.
Once a site has selected the creation of access roads, drainage infrastructure, and the Well pad starts. Grading of the site, excavation of drilling fluid pits, and the transportation of operating equipment take place.
5.2 Well Construction
Construction begins with the use of a drill rig. Operators hoist sections of drill pipe and drill bit—a configuration known as a drill string or column—and begin drilling the well. While the drill advances through the soil and into rock, drilling fluid is circulated through the hollow centre of the drill string to lubricate the bit and reduce the temperature.
Following well design specifications the drill is removed and casing (steel pipe) is set into place within the well. Subsequently, cement is pumped into the well filling the space between the outside of the casing and the well. This process is repeated with a smaller diameter drill string and bit, ensuring a sealed, reinforced well.

Figure 4. Brief Well diagram
5.3 Well Casings

Figure 5. Well casings
The casings installed into wells are designed with different purposes and have different names as follows:
Conductor Casing: Usually uncemented, this casing is designed to prevent dirt and rock from entering the drilled holed and is in the uppermost section of the well.
Surface Casing: Thinner but wider than the following layers of casing, it protects groundwater from contamination and equipment from blowouts.
Intermediate Casing: This layer of the casing is designed to reinforce the well against pressures found at intermediate depths. The intermediate casing may also be used to prevent groundwater contamination at these depths.
Production Casing: Located at the furthest depth, production casing accepts the flow of hydrocarbon resources into the well. This layer of casing contains perforations for hydraulic fracturing.
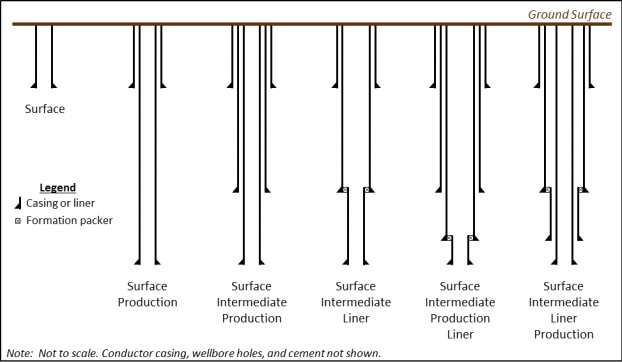
Figure 6. Layered Well casings and delineation
6 Fracturing Fluid
The fluid used to hydraulically fracture wells is an engineered mixture of water, proppant, and chemicals. This fluid is designed to fracture the geologic formations containing hydrocarbons and prop the resulting fractures open. Propping these fractures open facilitates the flow of oil and natural gas through the well.
6.1 Type of Fluids
Fracturing fluids are composed of 88% water, 10% proppant (quartz or sand), and less than 1% additives on average. Each producer uses slightly varying compositions of fracturing fluid. Over the decades of experimentation with hydraulic fracturing fluids, several types of fluid have been developed for application across a wide variety of settings.

Table 1. Fracturing Fluid Types
The most common fracturing fluids use water as a base.
6.2 Proppants
Fracturing fluids, while composed primarily of water, make use of sand, quartz, or synthetic materials to prop open micro-fissures within unconventional resource formations. Since the 1940s, proppants have been experimented with to discover which methods increase well production.
Several varieties of sands, glass, molten zirconia, aluminium pellets, ash, and other materials were tested. From these, three main materials emerged as productive and economical.
6.2.1 Rounded Silica Sands
Silica sand is a proppant material known for its inexpensive nature and availability. Though inexpensive, silica sand has a low crushing strength capable of operating up to 6,000 psi.
6.2.2 Resin Coated Sands
Silica sands may be coated with phenolic resins which are more resistant to crushing. When polymerized on-site, in the reservoir, these sands become resistant to the acidity of the environment. Once crushed by stress, the resin from these proppants acts as a filter, preventing clogging within the flow channel. If cured before injected into the well, these resins coated sands can prop open fractures reliably within the formation. These sands may endure environments between 6,000 and 10,000 psi.
6.2.3 Synthetic Ceramic Materials
At greater depths, stronger materials such as bauxite or ceramics are used to withstand extreme pressures while reliably propping fractures open. Heating, melting, or sintering bauxite, kaolinite clays, or other ceramic materials is the most common method of producing these proppants. They are capable of functioning in environments exceeding 20,000 psi and remain chemically inert.
7 Hydraulic Fracturing with Nanotechnology
Conceptually introduced to the scientific world by Nobel Prize-winning physicist Richard Feynman in the 1960s, nanotechnology has been pursued by academics, industries, and scientists alike. Fundamentally, the science of nanotechnology is the manipulation of atoms and molecules on the nanoscale.
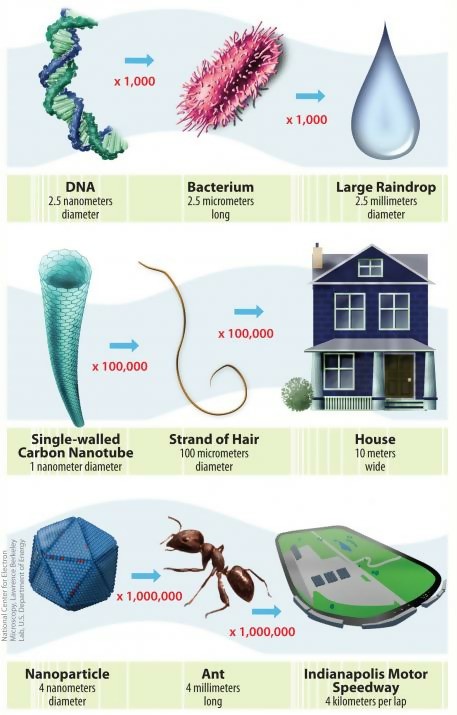
Figure 7. Nanoscale
Though used in many aspects of oil and gas exploration and production, nanotech has seen regular and increasing use for the last five decades in hydraulic fracturing. Nanoengineered coatings, fluids, proppants, and sensors are regularly integrated into the exploration and extraction of oil and gas from both conventional and unconventional resources.
Nanotechnology is particularly effective in extracting oil and natural gas from unconventional resources. Nanosensors allow incredibly precise evaluation of fracturing effectiveness in unconventional reservoirs, while nanocoatings enhance the durability, efficiency, and effectiveness of equipment, oil and gas infrastructure, and proppants. Nanosilica and nanocoated proppants—like those made by Oxane Materials in Houston, Texas—are extremely effective in their aim to increase the productivity of hydraulic fracturing operations.
8 Fracturing
Injecting hydraulic fracturing fluid into the well takes place once the well is completed and a wellhead is installed. The mixture of fracturing fluid components takes place on-site. Once mixed, the fluid is pumped into the well through wellheads and high-pressure pipes at rates necessary to exceed the structural strength of a target formation.

Figure 8. Three wellheads with high-pressure pipes connected
Well-casings are designed to withstand the tremendous pressure and temperature changes exerted by this process. As the formation is sufficiently fractured, oil and natural gas flow freely through the well; the process typically lasts about two weeks, relatively short compared to other operating phases of the well.
Once the well has developed enough microscopic fracture networks and the flow of resources is developed, the pressurized injection of fracturing fluids is stopped. Fracturing fluid flows out of the well over time and is stored on-site in excavated pits or steel wastewater tanks designed for this purpose.
9 Finishing A Well
Hydraulic fractured wells produce resources for years, often for decades (up to 70 years by some estimates). Since the ability to produce hydrocarbon resources from unconventional sources has dramatically improved in recent years, there is a lack of experiential data associated with the production lifespan of unconventional wells, though estimates like those above are reasonable assessments based on traditional Well production lifespans.
After a well has reached the end of its product lifecycle, the well is disengaged from transportation pipelines and other infrastructure. Sealing a well involves installing permanent cement or mechanical plugs in sections throughout the well. Between seals, these sections are filled with bentonite clay or drilling mud. Local regulations state the material requirements and minimum standards for plugs and seals.
Thoroughly sealed, the top of a well is capped with a welded steel plate. Finishing a well entails plugging the well, removing associated equipment and restoring the land to original condition, the final steps in the lifecycle of a production well.
10 Environmental Concerns
With the rising prominence of hydraulic fracturing, concerns have grown regarding several aspects of the process. Concerned citizens and critics alike have alleged the risk of contaminating groundwater sources, increasing methane emissions, potential seismic activity increase, and the use of large amounts of water to fracture a well as notable issues.
These issues bear kernels of truth worthy of investigation. Data gathered since the advent of modern hydraulic fracturing shows these concerns to be meritorious, albeit significantly mitigated and in some cases entirely reversed. These concerns have had a remarkable impact on the perception of ‘fracking’ as a wasteful and even harmful practice.
10.1 Ground Water Contamination Risk
Groundwater contamination is a possible, hotly debated, occurrence associated with hydraulic fracturing. Multiple barriers exist between hydraulic fluid and the possibility of contaminating groundwater. Beginning with well placement and design, all aspects of a hydraulic fracturing operation contain multiple fail-safes which stand between contamination and the environment.
Steel casings reinforced by cement form air-tight, multilayer enforced well structures designed to eliminate the possibility of micro-fractures due to pressure, temperature, and the passage time. These efforts are aided by the natural properties of unconventional resource traps or reservoirs, which contain hydrocarbons only as a result of the surrounding geology’s lack of permeability.
Taking these precautions reduces the contamination risk of hydrofracking to a minimum, yet there are occasional structural failures of wells or other difficulties which could cause leaking into the surrounding rock or soil. Despite these occurrences, the head of the EPA has gone on record stating there are no confirmed cases of groundwater contamination from hydraulic fracturing, though more than 2 million wells have been fracked in the last 70 years.
The administrator of EPA Lisa Jackson on hydraulic fracturing.

Table 2. Federal Laws and Hydraulic Fracturing
10.2 Emission Potential
There is some significant debate over the number of methane emissions produced by natural gas emissions as a result of hydraulic fracturing. The EPA’s current estimates show leakage rates just under 2% for methane within natural gas systems. From 2012 to 2015 methane emissions related to hydraulically fractured wells decreased a staggering 65%.
Requirements for leak detection and control equipment have contributed significantly to this improvement. Overall, due to the increased use of natural gas, U.S. emissions of CO2 are at 30 year low along with notable reductions of many other harmful emissions.
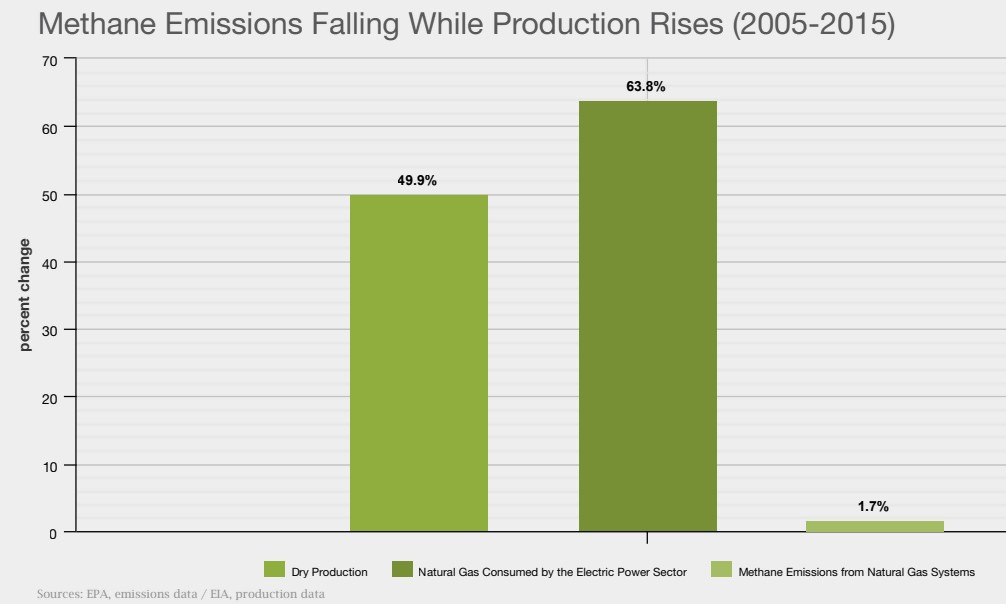
Table 3. Methane emissions 2005—2015
10.3 Seismic Activity
Though not directly related to the operations of hydraulic fracturing, the use of underground injection control (UIC) wells to safely dispose of wastewater has been linked to seismic activity in highly specific instances of geologic conditions.
A wide variety of human activities produce similar seismic activity when a closely located fault is under stress. The resulting slippage from the fluid injection can create felt, detectable earthquakes.
Induced seismicity is a complex, yet increasingly understood phenomenon that is addressed effectively on state levels. Ceasing injection-well operations or reducing injection rates and pressures quickly relieve seismic activity.
10.4 Water Use
As water comprises the majority of hydraulic fracturing fluid composition, the volume of water used to fracture the average well may seem excessive. The EPA’s extensive study on the use and impact of hydraulic fracturing fluid on the environment and water supply states that the average fractured well uses a median amount of 1.5 million gallons of water per well.
The same thorough examination of water use in hydrofracking conducted by the EPA consolidates dozens of case studies and data gathered from millions of fractured wells. Their conclusion states, with some exceptions, that hydraulic fracturing consumes a small percentage of water when contrasted to total water use at local, state, and national levels.
Despite this, the Western U.S. is more vulnerable to using local water resources for hydraulic fracturing. Rarely, areas conducting high amounts of hydraulic fracturing can reduce available drinking water. Areas that are afflicted by drought or other low water conditions must be carefully considered by producers; whose operations could reduce already minimal water supplies.
11 Summary
Hydraulic fracturing is a powerful and dynamic method of resource extraction, particularly when combined with horizontal drilling. These two technologies generated enough energy production and economic growth to fundamentally alter the economic dynamism of the United States on the world stage. Perhaps one of the most transformative technologies of the last several decades, expert crews and researchers continue to refine the efficiency, effectiveness, and safety of this remarkable technology.
12 Related Reading
U.S. EPA’s Study of Hydraulic Fracturing