1 Introduction
The production, transportation, and energy consumption through fossil fuels lead to many environmental issues today. Some of these issues include ozone depletion, acid rain, global warming, and climate change (Dincer, 2012:1954). Experts state that a rise in the average global temperature needs to be limited to 1.5°C above pre-industrial levels to avert some of the worst effects of climate change. This will be achieved when the Global carbon emissions decrease to zero by 2050 (IPCC, 2018). Various solutions to minimise the average carbon emissions have been developed, but the most promising is renewable energy and hydrogen. Increasing the use of renewable energy can help to decrease the amount of carbon dioxide emissions. Renewable energy depends on changing conditions and cannot always be supplied according to demand. Thus, it is mandatory to develop energy storage to store the generated renewable energy. One of the possible ways to store energy is through hydrogen. This report focuses on the value chain and the economic viability of hydrogen.
2 Hydrogen as an alternative
Hydrogen is the smallest and most abundant element in the universe. Hydrogen does not occur naturally but is always found in combination with other elements. Hydrogen can be produced by extracting it from a variety of compounds found in nature. Hydrogen extraction can take place in four different ways depending on the substance and the method used. Colours are used to classify the different processes (Israel et al., 2020:8).
Hydrogen production through processes contributing to carbon emissions is called brown or grey hydrogen. Brown hydrogen is manufactured from coal through a process revered to gasification. Gasification is when coal is reacted with super-heated steam to produce hydrogen and carbon monoxide. Grey hydrogen is produced from natural gas through steam methane reforming (SMR), where natural gas reacts with steam at high temperatures (Israel et al., 2020:8). Extracting hydrogen from fossil fuels is a pollution heavy process and contributes to 843 metric tons of carbon dioxide emissions every year. It is thus necessary to divert to cleaner methods of hydrogen extraction.
The zero carbon-emitting alternatives are called green or blue hydrogen. Blue hydrogen produces hydrogen using fossil hydrocarbons with adsorption carbon capture and storage (CCS). The carbon released during extraction is removed, minimising carbon emissions. Although this is a cleaner way of extracting hydrogen through fossil fuels, less than 0.4 metric tons of 69 metric tons of hydrogen use CCS (Israel et al., 2020:8). Green hydrogen is the extraction of hydrogen from water through electrolysis using renewable energy (Dincer, 2012:1955). This is the cleanest and most effective way of producing hydrogen from substances. However, Figure 1 shows that only 0.3% of the hydrogen produced is green hydrogen.
Figure 1 illustrates the production of hydrogen using water, coal, fossil hydrocarbons, and renewables. Hydrogen extracted from fossil hydrocarbons is responsible for 99% of the global hydrogen extraction, consuming 6% of the natural, 2% of the coal resources, and contributing to 2.2% of the carbon emissions. Conversely, zero carbon-emitting extraction methods are responsible for less than 1% of the produced hydrogen. The reason for this is that electrolysis is a costly process, and several challenges remain. Challenges include transportation, storage, and the lack of infrastructure. To ensure zero-carbon emission energy, much money and resources need to be invested in green hydrogen (Israel et al., 2020:9).
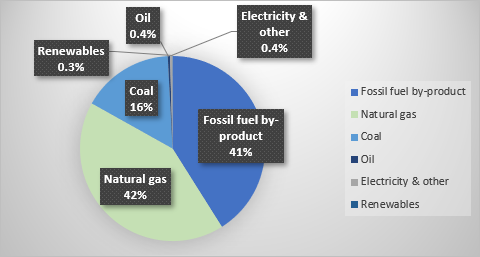
Figure 1-Global hydrogen production. Source: BofA GLOBAL RESEARCH
3 Green hydrogen in a hydrogen economy
The future of a hydrogen economy lies in green hydrogen. The energy used during electrolysis needs to be renewable to produce green hydrogen. This renewable energy can be solar energy, wind energy, hydropower, runoff waters, and fissionable materials. Green hydrogen is an important concept as it can be utilised in sectors and industries responsible for high carbon emissions. This includes the oil and gas, transportation, iron, and steel industry (Dincer, 2012:1955).

Figure 2-Value chain of green hydrogen. Source: Herbert Smith Freehills.
3.1 The production of green hydrogen through electrolysis
Water can be decomposed into hydrogen and oxygen molecules through electrolysis. Electrolysis is an energy-intensive process that requires renewable energy to produce hydrogen. Electrolysers consist out of an anode and cathode submerses in water. A permeable membrane separates the anode and the cathodes. An electrical current is passed through the electrolyser, causing the water molecules to split into oxygen and hydrogen. The oxygen and the hydrogen are separated, and the hydrogen is used to store the renewable energy (Israel et al., 2020:10). The energy required to extract hydrogen from water is higher than the energy released from hydrogen. Due to this property, hydrogen is used to store renewable energy (Barbir, 2004:661).
Conversely, the reversed reaction consumes hydrogen and oxygen and produces energy and water. Energy is produced in the form of a current and is said to be renewable. The energy can power a motor vehicle, add electricity to the grid, etc. There are different electrolysers, namely Alkaline, Proton Exchange Membrane (PEM), and Solid Oxide (Israel et al., 2020:10).
3.2 Alkaline electrolyser
Most electrolysers are based on Alkaline as an electrolyte. The electrolytes are submerged in an aqueous alkaline solution of 30% KOH. During electrolysis, charge flows between the electrodes and the current in the form of OH-. Consequently, hydrogen is formed at the cathode while oxygen is formed at the anode. A membrane separates the two reaction areas to ensure that the hydrogen and the oxygen do not mix (Artuso, et al., 2010:7956). This electrolyser is inexpensive but has a lower energy efficiency than PEM electrolysis (Israel et al., 2020:10).
3.3 Proton Exchange Membrane electrolyser (PEM)
PEM electrolyser uses a proton exchange membrane as an electrolyte. Protons pass through the PEM with the help of electroosmotic drag and form hydrogen at the cathode. Respectively, oxygen forms at the anode. Much research is currently conducted on PEM Electrolysers as it produces greater efficiencies, versatility, and higher power. The biggest barrier to overcome is the higher cost than Alkaline electrolysers (Litster & McLean, 2004:61).
3.4 Solid Oxide electrolyser (SOEC)
The electrolyte of the SEOC consists out of a solid ceramic material heated to 700°C to 900°C. Water and carbon dioxide are heated before entering the SEOC, and through electrolysis, hydrogen and carbon monoxide are formed. After that, hydrogen and carbon monoxide with a catalyst produces a synthetic fuel (Jensen et al., 2010:9544). Although SOEC is more efficient than PEM, it is still in a research phase and has not been performed on a large scale yet (Israel et al., 2020:10).
4 Storage of Hydrogen
Pure hydrogen can be stored in the liquid and the gas phase depending on the resources available. The large-scale storage of pure hydrogen is important for a hydrogen economy to function effectively. Hydrogen is already being stored in salt caverns beneath the ground on an industrial scale. Due to the lack of salt caverns and geological conditions, alternative storage methods are being researched. These methods are liquid hydrogen and compressed hydrogen, considering storage density, costs, and safety (Andersson & Grönkvist, 2019:11901).
4.1 Salt caverns/underground storage
Salt caverns are the most suitable method to store hydrogen underground as it is inexpensive and low on construction costs. This method also has high injections and withdrawal rates, low to zero leakage rates, and low risks of contamination. Apart from the numerous advantages, this method cannot be considered the main hydrogen storage solution. The reason for this is not all regions have the proper geological prerequisites. There exists a large natural gas vessel below the ground that is lined with a steel cylinder. This lined rock cavern is in Sweden and can store approximately 740 tons of hydrogen if stored under the same conditions as natural gas. It is possible to store hydrogen the same way, but further research is needed (Andersson & Grönkvist, 2019:11903).
4.2 Compressed hydrogen gas
A storage system that compresses gas consists out of storage compartments and compressors. Hydrogen is compressed and stored in metal containers. Metal containers are used despite the high investment costs. Metal containers ensure the purity of the hydrogen, stability of the containers, and independence of location. Compressed hydrogen gas in metal containers can be stored above and below the ground (Andersson & Grönkvist, 2019:11903).
Containers can be stored underground in cases where space is limited. Storing larger containers underground saves space, protects containers against weather, and insulates pure hydrogen gas. However, maintenance of underground containers is difficult and external coatings are needed to prevent corrosion. The same types of vessels used to store natural gas can be used to store hydrogen gas. The three main types of vessels include gas holders (storage pressures slightly above atmospheric pressure), spherical pressure vessels (storage pressures below 20 bar), and pipe storages (storage pressures below 100 bar) (Andersson & Grönkvist, 2019:11903).
For large-scale storage, pipe storages are the most suitable due to the higher storage pressure. A series of pipes are placed a couple of meters below the ground with the ends sealed. The length of a pipeline series can be several kilometres long, storing up to 12 tons of hydrogen per kilometre of pipeline. The construction of hydrogen pipelines is more expensive than that of natural gas. This is due to hydrogen embrittlement that negatively impacts the mechanical properties of steel materials (Andersson & Grönkvist, 2019:11903).
4.3 Liquid hydrogen
Hydrogen can be stored in the liquid phase through liquefaction. With liquefaction, very high hydrogen storage densities can be gained at atmospheric pressure. The biggest disadvantage of liquid hydrogen is the cost of liquefaction. This process is very expensive due to the extremely low boiling point of -253°C, and that hydrogen gas needs to be precooled through evaporating liquid nitrogen. In addition to the high costs of liquefaction, the capital costs of a liquefaction plant consume 40-50% of the total costs of liquefaction. Nevertheless, hydrogen liquefaction is a well-developed process used in the industry (Andersson & Grönkvist, 2019:11904).
Once the hydrogen has been liquefied, it should be stored in vessels designed to minimise liquid hydrogen evaporation. The evaporation of hydrogen leads to a loss in the energy used for liquefication and a loss in the hydrogen escaping through the vessel’s vents. The loss of evaporated hydrogen per day is known as the boil-off rate. The storage vessels are designed with two walls with a high vacuum between them to prevent heat transfer. Additional materials such as alumina-coated polyester sheets are also placed between the walls to prevent heat transfer due to radiation. The high degree of insulation in large spherical vessels can limit the boil-off rate up to 0.1% per day. The largest operated storage vessel stores up to 270 tons of liquid hydrogen. It is believed that even larger liquid vessels are possible (Andersson & Grönkvist, 2019:11904).
The boil-off of hydrogen is less concerning when the liquefaction plant and the storage are close in approximation. This is because the evaporated hydrogen can be injected back into the late stages of the liquefaction process and be converted into liquid again.
Although liquid hydrogen storage is more complex, it obtains a lower cost per weight of hydrogen stored than pressurised hydrogen on a large scale (Andersson & Grönkvist, 2019:11904).
5 Transportation of Hydrogen
Hydrogen can be transported in liquid and the gas phase.
5.1 Gaseous hydrogen transportation
Hydrogen gas can be transported through gas containers in tube tanks or through gas pipelines.
5.1.1 Pipelines
Gas pipelines can stretch for several kilometres transporting hydrogen gas from hydrogen plants to consumers. This type of transportation can have a high investment cost. Because of this fact, researchers are exploring the use of existing natural gas pipeline infrastructure to transport hydrogen gas (Moradi & Groth, 2019:12259).
5.1.2 Tube trailers
Researchers are in the process of designing a high-pressure tube trailer. These tanks can store up to 616 kg of hydrogen at 250 bars. This mass is 7% of the total weight of the high-pressure tube trailer. This method of transport is favourable as it does not require specific infrastructure. Furthermore, hydrogen loss in tube trailers is minor, and the compression cost is low. The compression cost can reduce up to 60% in comparison with liquid hydrogen transportation. In terms of safety, tube trailers undergo penetration tests, hydrostatic burst, cyclic temperature tests, leakage tests, and pressure tests. Some concerns include liner blistering because of saturation and decompression at 350 bar, the high cost of production, the small capacity of tube trailers, and the restriction of size and pressure due to regulations (Moradi & Groth, 2019:12259).
5.2 Liquid hydrogen transportation
Liquid hydrogen transport is economical if the demand is high (more than 500kg/day) and the travelling distance is not extremely far. This type of transport is known as cryogenic hydrogen delivery. Cryogenic tanks are used to transport liquid hydrogen from liquefaction plants to consumers. Hydrogen fuel stations are supplied with liquid hydrogen rather than gas hydrogen because of the higher capacities of liquid hydrogen transport (Moradi & Groth, 2019:12259).
6 Applications of Green Hydrogen
Hydrogen can be used in the transportation, industrial, energy, and agriculture sector. In transportation, hydrogen powers hydrogen fuel cells in trucks, cars, and trains. In the energy sector excess, renewable energy is stored as chemical energy in hydrogen. This can overcome the unstable and unpredictable energy supplied by renewable energy sources. Hydrogen can also be injected into gas networks for domestic use (Developing Green Hydrogen projects, 2021)

Figure 3-Schematic of the distribution of renewable energy through hydrogen. Source: Yang, Ma, Lian, Zhang & Pang, 2021:126830
6.1 Hydrogen fuel-cell
Hydrogen can act as a fuel in a hydrogen-powered vehicle. Using hydrogen as a fuel source can help implement renewable energy in the transportation market. Hydrogen fuel cells are proven to be more efficient and sustainable than electrical batteries (Dincer, 2002:273).
The first focus of transition is on fleet vehicles like transit buses. The reason for this is that depots will facilitate hydrogen as fuel, buses are a significant source of carbon dioxide emissions, and hydrogen-powered buses are expected to be low on maintenance costs. Hydrogen is pumped into a fuel cell bus, and compressed hydrogen is fed to the engine. The performance of the bus is the same as a diesel-fuelled bus with more advantages. These advantages are softer engines and less harmful emissions. Major automobile companies are also turning their attention to fuel cell technology. Most companies have already launched hydrogen-powered vehicles that are available for public purchasing (Dincer, 2002:273).
Fuel cells have numerous advantages. It can produce electricity efficiently without any moving parts. The temperatures at which electricity is generated are relatively low since no combustion is required. Without combustion or moving parts, the vehicle can go longer before going for a service. Fuel cells were proven to be safe, very reliable, and less prone to break. Also, fuel cells can help reduce carbon emissions as the energy used to produce hydrogen is entirely renewable energy (Dincer, 2002:274).
Fuel cells are based on the same principle as a battery, but it does not run down or need to be charged. Hydrogen reacts with oxygen in an electrolyte to generate a current between two electrodes. Therefore, it converts the chemical energy of hydrogen and oxygen to water, electricity, and heat. Oxygen is obtained from the atmosphere and hydrogen from a hydrogen fuel station. As long as hydrogen is supplied to the engine, electricity will be generated along with water and heat as by-products (Dincer, 2002:274).
Hydrogen reacts with oxygen in an electrolyte to generate a current between two electrodes. Fuel cells can be categorised based on their operating temperatures, namely low temperature and high-temperature fuel cells. Low-temperature fuel cells have a quick start-up time, a smaller volume, and lower weight than high-temperature fuel cells. Examples of low-temperature cells are PEM, alkaline, phosphoric acid, direct methanol, and unitised regenerative fuel cells. High-temperature fuel cells are more power-efficient than low-temperature fuel cells. These fuel cells produce excess heat, which is problematic in transportation. However, this excess heat is favourable in stationary applications (Dincer, 2002:275).

Figure 4-Schematic of the working of a fuel cell. Source: Dincer, 2002.
6.2 Green hydrogen in a smart grid
Renewable energy is integrated into the main energy grid through means of storage. Energy can be stored in hydrogen as chemical energy. The demand for renewable energy is increasing due to the environmental crisis caused by carbon emissions and the increasing prices of fossil fuels. Renewable sources supply unstable and unpredictable energy as it is dependent on numerous factors, like the time of day, weather, and the season. This can lead to an electricity shortage during peak usage. To resolve this problem, monitor, sensor, and communication systems should be implemented into smart grids. Furthermore, excess renewable energy can be stored in hydrogen. Storing excess renewable energy in hydrogen can overcome shortages during peak periods (Lin et al., 2020:20165).
Microgrids are small power systems that are an important development regarding smart grids. These girds are highly reliable and can supply electricity to local demands. They are comprised of a solar panel, a hydrogen fuel stack, and a tank that stores hydrogen. Microgrids can either be stand-alone or grid-tied (Lin et al., 2020:20165).
This sector still needs more research and resources to achieve zero carbon emission energy by 2050.
6.3 Hydrogen in existing natural gas networks
Hydrogen injected into natural gas networks can allow for indirect electricity transport and storage. The composition of the gas mixture can vary at different locations. A mixture can consist of up to 10% hydrogen in the volume of natural gas. If a natural gas refuelling system is connected to the network, this ratio can crop to 2%. More research is necessary to inspect the impact of the gas mixture on the pipe network (Abeysekera et al., 2020:992).
7 The Cost and Viability of Hydrogen
The two sectors developing at an immense speed are fuel cells in transportation and hydrogen injection in existing gas infrastructure. This is because major automobile companies compete to develop a hydrogen-fuelled vehicle at low cost and high efficiency. Hydrogen injection is a more cost-efficient way of adding hydrogen power to the grid.
7.1 Hydrogen cost development
Large industrial producers determine the internal Hydrogen prices in Europe. This price depends on the consumer’s location, which determines if the hydrogen is delivered in a gaseous or a liquid phase. This price is also dependent on the quality and the purity of the hydrogen. Transportation, storage, and distribution are important in hydrogen production and contribute to the total cost of hydrogen. In Europe, the main production method used is Steam methane reforming (SMR). As mentioned in Section 2, SMR produces carbon dioxide; thus, the produced hydrogen is classified as grey hydrogen. This is expected, as 99% of hydrogen production is through fossil fuels. Today the price of hydrogen varies between 10 and 60 €/kg of hydrogen, where 60 €/kg is the purist available hydrogen. The futuristic prices of hydrogen are calculated by analysing its production costs. As the cost of hydrogen decreases, the demand will increase. It is predicted that the demand will increase to around 750000 tons/year in 2030. Figure 5 illustrates the cost of hydrogen in the past and the predicted costs according to different studies (Jovan & Dolanc, 2020:3).

Figure 5-Cost of hydrogen from 2015 to 2030 (7. Financial viability_3)
7.2 Cost of hydrogen for household consumers
Hydrogen gas prices for household consumers are 6 €/kg. This price cannot compete with the price of natural gas at 1 €/kg. (https://www.euractiv.com/section/energy/opinion/what-is-the-real-cost-of-green-hydrogen/)
Researchers show that injecting hydrogen into exciting gas infrastructure is more cost-efficient than integrating hydrogen into main grids. The reason for this is costly grid upgrades and storage facilities (Erbach & Jensen, 2021:2)
7.3 Cost of hydrogen fuel
The cost of hydrogen fuel will mainly depend on production, transportation, and distribution. It is expected that hydrogen fuel costs will be high initially due to high production costs and low purchases (Jovan & Dolanc, 2020:3). In Germany, the hydrogen fuel price currently is 9.50 €/kg (one kilogram of hydrogen can fuel a fuel cell for 100 kilometres) (H2. LIVE: Hydrogen Stations in Germany & Europe, 2021). At the same time, the price of gasoline is 1.39 €/litre (Gasoline prices Germany 2021 | Statista, 2021). Although the price of hydrogen fuel is costly compared to gasoline, the hydrogen fuel prices are decreasing as the total consumers increase (Jovan & Dolanc, 2020:3).
8 Conclusion
Green hydrogen is a promising solution to zero-carbon emission energy. Since 99% of the hydrogen produced today is extracted from fossil fuels, a great deal of capital and resources is necessary to make green hydrogen the main production method. Although green hydrogen is more expensive than fossil fuels, the reduction in environmental impact, competition between major automobile companies, and decreasing hydrogen prices are making green hydrogen more attractive.