1 Background
1.1 Key Issues and Challenges
Key issues and challenges ahead for investors, policymakers, regulatory commissions and clean energy program administrators that contribute to uncertainty in forecasting future pathways include largely external factors. At the same time, financing and policy choices, as well as regulatory and program practices, also heavily influence energy transition pathways [1].
- The evolving generation mix and current economics of supply-side options are changing the value proposition for energy efficiency resources. The result is a greater focus on time-varying value (e.g., to help meet peak system demand) and locational value (e.g., for load relief on distribution systems), more emphasis on controllable loads (e.g., to increase system flexibility), and more interest in bundling demand-side options such as energy efficiency, demand response, distributed generation and storage, and electric vehicles in order to provide various grid services.
- A turbulent global economy and shifting policy objectives: this complicate forecasting of future clean energy needs;
- State leadership drives institutional frameworks for clean energy and energy efficiency. Clean energy resources have distinctive characteristics that require state regulatory commissions to establish an institutional framework for effective oversight of utility customer-funded programs.
- Achieving higher market penetration rates. This includes targeting and reaching traditionally underserved markets (e.g., small commercial, multifamily, rental housing, non-owner-occupied commercial buildings) in far greater numbers than current practice. Program administrators also need to design new, innovative programs that offer different strategies and services that are attractive to customers.
- Achieving deeper savings. Solutions may include strategic energy management programs for industrial customers, greater reliance on building and industrial controls, programs that focus more on upstream/midstream market interventions (e.g., incentives to retailers and vendors), and programs that combine technical assistance with incentives and financing (e.g., green bank, on-bill financing)
Addressing the subjects mentioned above will require stakeholders’ financial commitment to the public and private sectors to fire up change in how we use and produce energy. Although such changes provide tremendous economic opportunities for stakeholders at all levels, the current lack of knowledge, the need for suitable financial products, and the barriers to making initial investments are significant constraints to scaling and implementing clean energy solutions.
Innovative institutions use the extensive knowledge of clean energy finance to address these financial barriers and increase access to capital by developing groundbreaking financing policies and mechanisms.
1.2 What is a Green Bank?
There is consensus that financing is an essential tool for mobilising capital to encourage a successful energy transition [2]. The recent surge of energy justice policies pushes for equitable access to sustainable technologies, benefits, and affordable energy for all.
There exist international nonprofits that are in the business of creating and operating green banks around the world. Specifically, green banks are dedicated finance institutions, often public or nonprofit, that connect clean energy projects with capital. Green banks are not banks in the sense that they take deposits; instead, they ensure that financing, which is the lifeblood of any clean energy project, is readily available to green initiatives in their jurisdiction.
Often, green banks tackle the toughest problems in the industry, serving as the glue that can hold together an otherwise unfinanceable project in the eyes of a part of the private sector. Green banks are not competing with private capital; instead, they are in the business of growing the pie of the financing market, building it out, of using limited public resources to maximise overall investment in the clean energy economy in their jurisdictions [3].

Figure 1. Green Banks are designed to fill the gaps left by other financial institutions and the market. Source: https://www.cocleanenergyfund.com/green-banks-101
The trend is to look to financing to address social and economic disparities through six proposed principles to ‘just’ energy finance: (1) affordability; (2) good governance; (3) due process; (4) intra-generational equity; (5) spatial equity; and (6) finance resilience. These six principles rest upon the principles of energy justice that address distributional, procedural, and recognition justice.
Green banks and community development financial institutions (CDFIs) are well positioned to play this role, having already expanded access and mobilised private capital by utilising public funds to create loan loss reserves, credit enhancements, and other tools to lower the cost of capital.
2 Modelling and Basic Contracts
2.1 Clean Energy Business Models
Business models structure how business investments are designed, implemented, and managed and incorporate critical financing, service, and monitoring features. The most appropriate business model for a given project will depend on local conditions, the financial and regulatory environment, and the institutional framework and support mechanisms in place.
The scale and purpose of the project or service must be well defined, together with the end consumers. Business models are not set structures; each must be adapted to the local circumstances and risk profiles of the selected project.
Business models can be broadly categorised as follows:
- Ownership models, which focus on financing and risk mitigation concerns; and
- Service models focus on providing specified services and highlight different operation and maintenance methods.
In practice, most real-world business models are hybrids, combining elements of various types and approaches. For example, a utility or a private company may develop and own a mini-grid system, operated and managed in turn by a community-based organisation. In contrast, a private maintenance company provides technical backup and support services.
The following considerations bear on the choice of the appropriate business model: (i) product or service considerations, (ii) the scale of the project, (iii) the consumer, and (iv) the regulatory environment.
As a rule, projects are assessed mainly in terms of their financial feasibility. However, renewable energy and energy efficiency projects may also have substantial, nonquantifiable or non-monetisable public good benefits.
Rural electrification projects, for example, may contribute to the following benefits:
- Labour savings (from not having to collect wood);
- Reduced deforestation (from less reliance on fuel wood and charcoal);
- Health benefits (from reduced indoor air pollution and improved water and sanitation services);
- Education benefits (from improved lighting in schools and residences and better study conditions); and
- Reduced poverty (from agricultural and industrial productivity improvements).
Such benefits would be difficult to reflect in electricity tariffs for consumers. The appropriate business model may therefore need to provide for some form of public subsidy or recognition that tariffs would at most cover only the cost of supply.
2.2 Public–Private Agreement
Public–Private Partnership schemes usually provide a service or exclusivity in the right to “own” specified public infrastructure, such as a highway section, over a given time period and the right to impose a toll for its use. A PPA involves a contract between a public sector authority and a private party. The private party provides a public service (e.g., electricity supply) and assumes a substantial amount of the financial, technical, and operating requirements. The main purpose of a PPP is to allocate the tasks and risks to those parties best able to manage them, notably the private sector partners.
Depending on how the PPA contract is structured, the cost of using the service can be borne exclusively by the service users (no taxpayer or public participation) or the government, or by both in some blend of these opposite approaches. Government contributions to a PPP may also be in kind, for example, the transfer of assets or land. Alternatively, the government may support the project by providing revenue subsidies through favourable taxes or other means.
Standard PPP models are:
- build–own–operate–transfer (BOOT) model;
- build–own–operate (BOO) model; and
- build–own–transfer (BOT) model.
Because of their complexity and high overhead costs, BO(O)T forms of PPP are usually favoured for large infrastructure projects. The special-purpose or project company is the central administrative and operating entity handling all contracts for funding with equity and debt investors. It also manages the construction and operation and maintenance (O&M) contracts (Figure 2), as well as the billing of end users. This type of business model is suitable for both conventional and renewable energy projects.

Figure 2. New ships for ever-larger offshore wind farms installation. Source: https://ulstein.com/news/covering-the-entire-life-span-of-offshore-wind-farms
2.3 Multiparty Ownership
A multiparty ownership model is particularly suited to energy projects with high technical complexity or multiple separate elements, such as biogas production and power generation. Multiparty ownership models are frequently combined with user co-op models so that the projects are part public and part private. This ownership model can be a good option for multipurpose renewable energy projects, such as community-based biogas digester projects.
Critical aspects of the multiparty ownership model, as applied to an energy project, are the following:
- Renewable energy or energy efficiency projects may be technically complex and have high capital costs, requiring unique models (to achieve economy of scale).
- In the case of biogas digester generation systems, the power-generating equipment is funded and installed by the utility, and the digester is owned and maintained by a third party (energy service company, user cooperative, or other entity).
- Funding is provided by a third-party installer or an outside source, freeing the farmer from any major liability. The equipment is installed at the farmer’s site.
- Revenues from the sale of the biogas to the utility are used to repay debt and interest.
- Implementation: biogas systems, micro- or mini-grid systems
- Benefits: low risk for the farmer; can incorporate donor funding for rural electrification
- Disadvantages: high technical risk (particularly if a third-party maintenance company does not properly support the farmer)
2.4 Dealer Credit
The dealer credit business model is still another variant of the ownership business model, where the equipment or system supplier provides the initial credit for the system. “As a ‘one-stop-shop’, the micro-finance institution (MFI) and the energy/technology company promote simple and standardised (accredited) energy products (e.g., solar photovoltaic systems), together with loans. The micro-financing function includes the collection of down payments, monthly instalments and system/capital recovery in case of default. The technology function includes marketing, installation, customer training and after-sales service” [4]. Ownership of the systems is transferred to consumers at the end of the loan repayment period.
There are two main forms of the dealer credit model: in the “one-hand” model, a single company provides both the technology and the financing, and in the “two-hand” model, the technology company and the microfinance institution (MFI) are separate entities but work closely together in a long-term partnership. The two-hand form makes it easier to diversify and customise energy products, but at the additional risk that the MFI may need to take over the project if the technology company fails to deliver proper services or product guarantees essential for loan repayment.
2.5 User Cooperative
The service-based business model is focused on providing a product or service to the end user. The service is provided by an energy service company (ESCO), which can be a private or public utility, a cooperative, a non-government organisation (NGO), or a private company.
A user cooperative business model involves establishing a nonprofit community organisation owned and managed by its members. Projects are funded by member contributions, with or without outside private or public support [5].
The cooperative manages all administrative and operational functions, including the installation, maintenance, and safe operation of renewable energy or energy efficiency projects, as well as financial management and payments between users, contractors and operators, and the cooperative. The tasks are usually performed by managers selected from among the members. As the managers may be volunteers, a lack of commitment and appropriate management skills may hamper efficient management.
A complete business plan should address the following:
- Community engagement and leadership. Community members, particularly users of renewable energy or energy efficiency measures, should be involved early in the planning process.
- Clearly defined ownership and maintenance responsibility. Rural electrification projects involving user cooperatives often have mixed ownership structures, with public and private entities financing and owning different parts of the system.
- Appropriate tariffs and collection arrangements. Tariffs should be set high enough to cover ongoing maintenance and operating costs, considering the variations in O&M costs with changes in system load. Tariffs must also be affordable to the members
2.6 Energy Performance Contracting
This business model is widely used in projects designed to improve energy efficiency. The ESCO receives performance-based remuneration, determined as a fixed portion of energy savings against a predefined baseline consumption of energy by the consumer. There are two primary types of energy performance contracts: guaranteed savings (consumer financed) and pay as you save (ESCO financed).
In the guaranteed-savings variant, the ESCO analyses the consumer’s needs and recommends the appropriate energy efficiency equipment and other measures. Once the consumer and the ESCO agree on the actions, the consumer provides financing for the measures (either through self-financing or a bank loan). At the same time, the ESCO implements the measures and shares in a portion of the guaranteed energy savings. In the pay-as-you-save model, the initial capital cost is financed by the ESCO, which recovers the costs through monthly surcharges. Both variations allow ESCOs to develop innovative programs that guarantee a level of energy savings for the consumer.
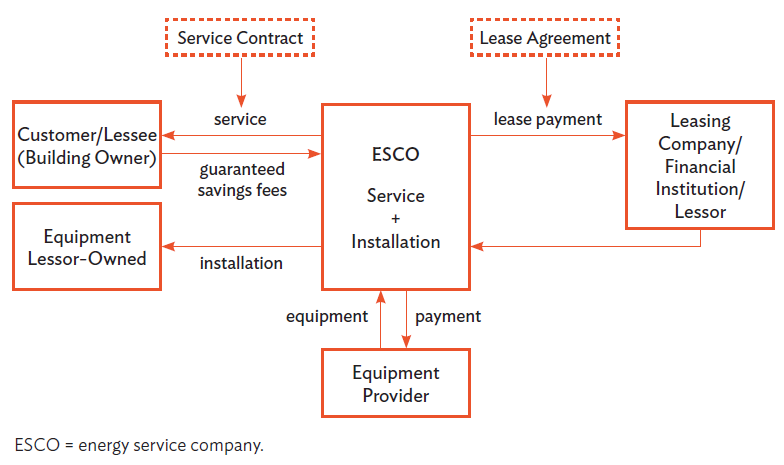
Figure 3. Energy Performance Contract: Pay-as-You-Save Relationship Diagram. Source: https://www.adb.org/
3 Solar Economics
3.1 Generalities
Solar facilities are generally characterised by high first costs and low operating expenditures. Thus the fundamental economic problem is comparing an initial known investment with estimated future operational costs. Most solar energy processes require an auxiliary (i.e., conventional) energy source so that the system includes both solar and conventional equipment; a combination of the sources is responsible for meeting the annual loads. In essence, solar energy equipment is bought today to reduce tomorrow’s fuel bill.
The cost of any energy delivery process includes all of the hardware and labour involved in installing the equipment, plus the operating expenses. Factors that may need to be considered include interest on money borrowed, property and income taxes, equipment resale, maintenance, insurance, fuel, and other operating expenses.
Finding the least expensive way to meet the requirement for energy can be seen as the goal of the economic analysis, considering both solar and nonsolar alternatives. For solar energy processes, the problem is determining the size of the solar energy system that delivers the lowest cost combination of solar and auxiliary energy.
3.2 Cost of Solar Systems
Investments in buying and installing solar energy equipment are important factors in solar process economics. These include the delivered price of equipment such as collectors, storage units, pumps and blowers, controls, pipes and ducts, heat exchangers, and all other equipment associated with the solar installation. The installation costs must also be considered, as these can match or exceed the purchase price. Also included are the fees of structures to support collectors and other alterations made necessary by the solar energy equipment.
Under some circumstances, credits may be taken for the solar process if its installation results in a reduction in costs; for example, a collector may serve as part of the weatherproof envelope of a building, eliminating the need for some of the conventional siding or roofing.
Operating costs associated with a solar process include the cost of auxiliary energy, energy costs for the operation of pumps and blowers (often termed parasitic energy, which should be minimised by careful design), extra real estate taxes imposed on the basis of the additional assessed value of a building or facility, interest charges on any funds borrowed to purchase the equipment, and others.
There may be income tax implications in the purchase of solar equipment. In the United States, interest paid on a mortgage for its purchase and extra property tax on an increased assessment due to solar equipment are both deductible from income for tax purposes if the owner itemises their deductions. States may allow similar deductions.
The income tax reduction associated with these payments depends on the owner’s tax bracket and serves to reduce the cost of the solar process [6]. Equipment purchased by businesses has other tax implications. Income-producing property and equipment may be depreciated, resulting in reduced taxable income and thus reduced income tax. But the value of fuel saved by using solar equipment is effectively reduced because a business already deducts the fuel cost from its income for tax purposes.
Consequently, when trying to understand the financial aspects of a solar system design, it is common to state the impact of available incentives as a separate item. In the United States, state-by-state incentives can be found in the “Database of State Incentives for Renewables and Efficiency” [7] by the North Carolina Clean Energy Technology Center. Some states have dozens of policies and incentives, while others have hundreds.
3.3 Solar Savings
As outlined by Beckman et al. [8], the concept of solar savings is helpful. Solar savings are the difference between the cost of a conventional system and a solar system. (Savings can be negative; they are then losses.) In equation form, it is simply:
In this equation, evaluating costs common to both the solar and the nonsolar system is not necessary. For example, the auxiliary furnace and much of the ductwork or plumbing in a solar heating system are often the same as would be installed in a nonsolar system. With the savings concept, estimating the incremental cost of installing a solar system is only necessary. If the furnaces or other equipment in the two systems are different, the difference in their prices can be included as an increment or decrement in the cost of installing a solar system. Solar savings can be written as:
Analogous equations can be written for income tax savings. They are, for a non-income-producing system, taking into account the effective tax rate and the incremental property tax, and, for an income-producing design, property tax, fuel expense, maintenance and insurance, parasitic energy costs, and depreciation. Fuel saved is a negative tax deduction since a business already deducts fuel expenses; the value of fuel saved is taxable income.
3.4 Levelized Cost of Electricity
The Levelized cost of electricity (LCOE) is perhaps the most commonly referenced metric of PV system economics. In simple terms, the LCOE gives a levelised (average) cost of electricity generation over the asset’s life. The LCOE is an analytical tool that can be employed to compare technology choices when different scales of investment or operation exist, thus facilitating an “apples to apples” comparison of technologies. For example, the LCOE could assess the cost of energy generated by a PV power plant with regards to that of a fossil fuel generating unit or another renewable source.
The calculation for the LCOE is the net present value of the total life‐cycle costs of the PV project divided by the quantity of energy produced over the system’s life:
The above LCOE equation can be disaggregated for solar generation as follows:
The costs include the initial capital investment and the lifetime operating expenses, less the end‐of‐life value discounted into the present value. The lifetime costs are divided by the lifetime energy production to derive the average cost of electricity.
When evaluating the LCOE and comparing other commonly known costs per kWh benchmarks used in the PV industry, it is important to remember that the LCOE is an evaluation of levelised life‐cycle energy costs and not the price of energy established under power purchase agreements (PPAs) or by feed‐in‐tariffs (FITs).
A Power Purchase Agreement contract is an agreement between the party that generates the electricity (the seller) and the party that is looking to purchase the electricity (the buyer). In the case of solar, the PPA contract generally covers a duration of 10, 20, or 25 years. A PPA provides the contracted annual revenue stream, which can support the financing of a solar project when no FIT exists.
A feed-in tariff is an economic policy created to promote active investment in and production of new energy sources. Feed-in tariffs typically use long-term agreements and pricing tied to production costs for renewable energy producers. The long-term security of a 10–25 year FIT contract provides a solar power system with the revenue needed to secure financing.
These may differ substantially from the LCOE of a given PV technology, as they may represent different contract or incentive durations, the inclusion of incentives such as accelerated depreciation or tax benefits, financing structures, and in some cases, the value of time‐of‐day production tariffs. One might consider LCOE to be similar to an automobile’s nameplate average fuel efficiency versus what a driver actually realises, based on their driving patterns (analogous to a FIT or PPA).
A system LCOE may be divided into the five key high‐level drivers listed in Figure 4: cost of capital, system life/residual value, plant energy production, annual operating costs, and PV system cost. Under these five categories, dozens of variables influence LCOE, and each can have a material influence on system economics.

Figure 4. PV LCOE drivers. Source: Reinders, A., Verlinden, P., Van Sark, W., & Freundlich, A. (2017). Photovoltaic solar energy: from fundamentals to applications. John Wiley & Sons.
4 Practical Cases of Clean Energy
4.1 The National Clean Energy Fund in India
India ranks third in terms of energy investments, but more needs to be done to meet its objective of producing renewable energy [9]. One of the main obstacles to such a transition is securing the money needed to meet the ambitious goal of producing 175 gigawatts of renewable energy by 2022. The issue is exacerbated by a lack of budgetary funds coupled with challenges in securing private finance for the industry. Yet, renewable energy financing in India reveals some fascinating evolution and patterns. Various incentives and instruments exist in the Indian market to support renewable energy financing. One is the National Clean Energy and Environment Fund (NCEEF).
NCEEF is one such funding mechanism available to support, among other things, renewable energy financing in India. The fund was created to support entrepreneurial ventures and research in clean energy technologies by mobilising funds through a cess on coal of INR 400 per ton known as Clean Environment Cess. The fund has been operational since 2011–12 and is primarily aimed at mobilising additional resources to support clean energy development.
Part of the fund gathered from the NCEEF is utilised by IREDA to lend to banks at a 2% rate so that this money can be lent to various renewable energy projects at a concessional rate of interest not exceeding a 5% rate. The budgeted estimates show that several thousand million Indian Rupees have been allocated to the Ministry of New and Renewable Energy (MNRE) under various heads to support renewable energy development in the country.
However, there exist several concerns with the fund. First, the renaming of the fund from ‘Clean Energy Cess’ to ‘Clean Environment Cess’ is a clear reflection of broad-basing the use of the fund for multiple purposes—essentially diverting the money for purposes not meant for. For instance, a recent assessment carried out on NCEEF shows that an increasing amount of the fund is diverted toward projects other than renewables [10]. The fund lacks appropriate monitoring mechanisms to oversee whether the financed projects have achieved their goals. There have been concerns with the allocation of resources for different projects as well as the operationalisation of the fund. It is argued that the cess has created a mess in utilising the fund and diverting it to areas that were not prioritised during the design of this funding scheme.
4.2 Connecticut Green Bank
Among the states that have created climate change mitigation banks, the longest-running and most prominent are in Connecticut and New York. Though structured somewhat differently— New York’s is a state agency, while Connecticut’s is quasi-public—the aim of both is the same: promote clean energy investment. Each institution grants similar lending products to finance climate-based initiatives and projects.
The first green bank in the US is the Connecticut Green Bank. By making clean energy finance available and affordable for homeowners, companies, and institutions, it is fostering a robust market to hasten Connecticut’s adoption of green energy. Its goal is to use the minimal public funding it receives to draw in large amounts of private capital to speed the deployment of sustainable energy inside the state. By doing this, it aims to combat climate change, increase energy security, and achieve economic success.
The Connecticut Green Bank received the renowned Ash award in policy innovation, also known as the “Nobel of policy,” from the Harvard Kennedy School of Government on July 12, 2017, in recognition of its contribution to “sparking the green bank movement.” The entity used financial structuring to attract private capital to clean energy projects. Back then, the bank’s management team had to carefully evaluate how various funding models would affect their aim to boost private investment in clean energy, produce jobs in Connecticut, advance energy security, and combat climate change. For example, the bank allows homeowners and businesses to rent solar panels—investments that might be commercially flawed without government guarantees or more accessible credit. Acknowledging their early success, other states and municipalities are on track to adopt their own—D.C. being the latest attempt.
Connecticut green bank’s investments have increased at a decreasing rate, while the private share it attracts continues to grow. This makes it possible for the bank to become self-sufficient because it does not call for additional taxpayer funding and the interest payments on its loans are sufficient to pay for ongoing projects. The total public stock from Connecticut’s bank has grown at a rate of approximately twenty per cent per annum, while private contributions have augmented by about thirty per cent.
Connecticut’s bank has attained a mobilisation rate of 8.7 in 2019, i.e. $8.70 in private investment was made for every $1 in public deal. One study has suggested that a national climate bank could reach one trillion dollars invested over thirty years, with only a thirty-five-billion-dollar public investment [11]. New York’s bank hasn’t witnessed this growth, but this could be for a variety of reasons—it’s newer, less focused on environmental issues, or simple swings in investment year-to-year.
4.3 RETScreen Portfolio Analysis
RETScreen clean energy management software can be applied for numerical analysis, such as energy generation and efficiency, emission reductions, prices, financial viability and risk of various types of renewable energy and energy-efficient technologies (RETs), based on a large database of benchmark parameters. This simplifies initial assessments and brings streamlined processes that enable designers, funders, regulators, etc., to decide on future clean energy initiatives.
In RETScreen’s portfolio analysis, we can be looking at multiple projects and how to take several of these and analyse them as a group. For example, we have several buildings, say schools, as part of a school board or manufacturing facilities for an industrial company, where we can do monitoring, verification and performance analysis.
We can aggregate all of these performance analyses, including the energy consumption prediction and the regressions, and look at them through the lens of a portfolio for a group to compare and contrast them, one against the other. We could also, for example, have several power projects as part of the power generating portfolio or maybe have several energy efficiency projects either planned or in progress on multiple buildings. We could analyse all of these together to tease out which projects are the most promising or, conversely, which are the most at risk.
There could be a series of individual measures, so we could have a portfolio of all the initiatives we have a stake in and compare them either from the performance standpoint or from their financial viability on a feasibility standpoint. The software can analyse certain energy efficiency projects and look at the pre-tax internal rate of return on equity per facility.
Financial analysis is typically provided as a final step of a pre-feasibility study. Some parameters are to be defined, such as inflation rate, project lifetime, debt ratio, duration, and interest rate. Thus, in the last section of the feasibility analysis table, the financial variability of parameters is calculated for each. User-defined costs usually include O&M for the project lifetime or labour force for the installation. Incentives could be introduced if available for the specific project.

Figure 6. Financial analysis chart (screenshot using RETScreen). Source: https://www.mdpi.com/2071-1050/12/5/2062/htm
The software gives four outcomes for evaluating financial viability:
- the actual interest yield provided by the project equity through its lifetime (before applying taxes) is represented by the pre-tax internal rate of return (IRR) on equity in percentage. The IRR on equity is calculated with the pre-tax yearly cash flows and the project duration. To clarify its meaning, it can be linked to the return on investment (ROI) or the return on equity (ROE). IRR on equity is used by the organisation as a comparison to the company IRR and to decide if the project investment is convenient or not. The IRR estimate may become inaccurate for a venture that requires cash injections during its lifetime, with amounts that are coincident with the project’s annual earnings.
- The pre-tax internal rate of return (IRR) on assets (%) is defined as the actual interest yield offered by the project assets over their lifetime (pre-tax). It can be resumed to the return on assets (ROA) meaning.
- the simple payback formula (in years) considers the expected initial costs, the total annual expenditures (excluding debt payments) and the total yearly income and margin. This indicator expresses how long it takes for a particular power plant or energy conservation measure to cover its initial cost. The simple payback method evaluates the desirability of investment: if the time to cover the initial cost is short enough, the investment could be considered opportune. This index could be used to compare different projects’ profitability. Although this indicator is of secondary importance for assessing investment risk, particularly given that it ignores crucial factors like the impact of inflation over the course of the project, it might be helpful for small businesses that should prioritise projects with quick paybacks even though their IRR is lower than those of other projects.
- the equity payback, which represents the time it takes for the power plant owner to cover its own initial investment (equity) out of the project cash flows generated. Equity payback takes into account the cash flow of the enterprise from its start as well as the equity (debt level) of the business, which allows it a better time measure of the value of the project than the previous simple payback indicator. The model uses the year number and the accumulated post-tax cash flows to measure this value.
As a result, financial feasibility is provided in Figure 6, together with the cumulative cash flow graph. In this allegorical case, less than one year is needed to reach the equity payback [12].
5 References
[1] https://www.iiec.org/key-activities/clean-energy-finance
[2] Hall S, Roelich KE, Davis ME, Holstenkamp L. Finance and justice in low-carbon energy transitions. Appl Energy 2018. https://doi.org/10.1016/j.apenergy.2018. 04.007
[3] https://nationalcleanenergyweek.org/
[4] Rolland, S. 2011. Rural Electrification with Renewable Energy: Technologies, quality standards and business models. Brussels: Alliance for Rural Electrification.
[5] Asia-Pacific Economic Cooperation (APEC) Energy Working Group. 2009. Successful Business Models for New and Renewable Energy Technology Implementation in APEC. Final Report.
[6] Duffie, J. A., Beckman, W. A., & Blair, N. (2020). Solar engineering of thermal processes, photovoltaics and wind. John Wiley & Sons.
[8] Beckman, W. A., S. A. Klein, and J. A. Duffie, Solar Heating Design by the f-Chart Method, Wiley-Interscience, New York (1977).
[9] https://www.adb.org/publications/green-energy-finance-india-challenges-and-solutions
[10] CSE. 2017. Using the National Clean Energy Funds to Clean Coal Power Plants. Policy Brief, Centre for Science and Environment. http://www.cseindia.org/ userfiles/NCEF.pdf
[11] http://coalitionforgreencapital.com/wp-content/uploads/2019/09/1T-investment-white-paper.pdf
[12] Argentiero, M.; Falcone, P.M. The Role of Earth Observation Satellites in Maximizing Renewable Energy Production: Case Studies Analysis for Renewable Power Plants. Sustainability 2020, 12, 2062. https://doi.org/10.3390/su12052062