Biological wastewater treatment utilizes the natural micro-organisms present in the waste streams to break down complex organic matter to cellular matter of bacteria, and thus effectively treating the water to remove the carbonaceous matter. Bacteria are the predominant group present in wastewater of which the E.Coli group is the most prevalent in sewage. Bacteria can adapt to the environment they are exposed to and based on the type of carbonaceous source, they will adapt their enzymatic system to suit the available food source which is referred to as the acclimatization phase. Treatment facilities rely on the bacteria being in the exponential growth phase, where the maximum amount of carbonaceous matter is absorbed for growth to ensure the treated water is of the best possible quality.
1 Cellular Energy Generation
Bacterial cells generate energy through the means of respiration (oxidative catabolism) or fermentation (fermentative catabolism), by taking electrons from the oxidized substance, which can be organic or reduced inorganic compounds. This is achieved through complicated biochemical reactions with the help of enzymes to transfer the electrons to another inorganic compound which is referred to as the electron acceptor. The most relevant electron acceptors are listed in decreasing order of energy release: oxygen (O2), Nitrate (NH3–), Sulphate (SO42-) and Carbon Dioxide (CO2). Depending on the type of micro-organisms present, they will utilize the electron acceptor present in the media with the highest energy release to allow for maximum growth of the micro-organisms. This results in the predominant metabolic pathway utilizing the oxygen present in solution, which is referred to as aerobic conditions. Once all the oxygen is consumed, micro-organisms present that are capable of utilizing nitrates start reducing it to nitrogen gas as part of the denitrification cycle. This is typically the transition zone referred to as anoxic conditions, where all oxygen has been consumed, but there is still the presence of nitrates. Once all the nitrates are consumed, full anaerobic conditions occur where the only electron acceptors present are sulphates and carbon dioxide. Sulphates are reduced to sulfides which are typically present as H2S, associated with the foul odour related to sewage treatment, and carbon dioxide, which can be reduced to methane.
Because the aerobic reactions release more energy, these organisms can reproduce faster than their peers and also makes the treatment process more effective at organic matter removal. The higher reproduction rate results in increased sludge formation, which is more efficient in organic matter removal from solution. Anaerobic reactions might not release the maximum amount of energy. Still, the final product compounds may have other advantages such as the possible precipitation of metal content as sulfide minerals, or the production of methane gas which can be recovered as an energy source. Depending on the source of wastewater that requires treatment, and the chemical composition of the water used as a transport medium, there may be unique opportunities available which would determine the best treatment process.
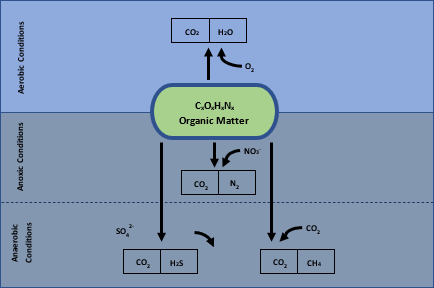
Figure 1: Various electron donor pathways for biological organic decomposition
2 Conditions Affecting Bacterial Growth
This article will describe the various processes related to wastewater treatment and how treatment facilities are optimized to achieve optimal carbonaceous matter removal. Understanding these parameters is crucial to operating wastewater treatment facilities, and how to manipulate them to achieve compliant discharge when there are changes in the feed water quality. By adjusting the operating parameters, the organic matter removal from sewage can be optimized to be achieved either through oxidation of organic matter (aerobic conditions) or the fermentation of organic matter (anaerobic conditions). For the organic matter to be utilized by the bacteria, it needs to be present as readily biodegradable, which is usually the case in domestic wastewater treatment. The larger compounds with more complicated chemical structures need to be broken down before they can be effectively utilized. This is achieved by exoenzymes present within the bacterial cell walls and referred to as hydrolysis. This process does not consume energy, but the complex compounds are transformed into smaller molecules which can be more readily consumed by the bacteria.
2.1 Carbonaceous Materials
To ensure growth and propagation of the microbial population, an appropriate substrate is required as a feed source. A substrate is referred to as a mixture of essential nutrients such as nitrogen, phosphorous, carbohydrates, fats, vitamins and minerals. This mixture is typically referred to as the feed carbonaceous substances and measured as biological oxygen demand (BOD). This is done by a standardized test which measures the cumulative oxygen concentration consumed after five days of incubation which is referred to as BOD5. During 5 days it is assumed that all the carbonaceous matter will be oxidized by the micro-organisms present under aerobic conditions, leaving just the nitrogenous compounds for final oxidation if the time is continued. In some cases, the oxidation of nitrogenous compounds can take place before five days are over, and in then adjustments would need to be made, which is why BOD test results can vary greatly.
The carbonaceous source must consist of a specific ratio of components critical to the growth of the inherent bacteria. In some cases where a specific element is deficient, it will be required to be dosed supplementary, but the guideline ratio for nutrients is:
BOD : N : P = 100 : 5: 1
Because wastewater treatment facilities rely on microbial cells being in the exponential growth phase, either one of the critical nutrient concentrations can be a rate-limiting factor and affect the efficiency of the facility.
2.2 Temperature
As previously stated, E.Coli is the predominant microbial organism present in sewage water and is referred to as a mesophile, which has peak growth in the range of 20°C to 40°C. Staying within this range, the microbial activity of a mesophilic bacteria can be described by the following equation (E.A.R. Ouano, 1981):
Equation 1
This equation indicates that the effect of temperature is exponential, and hence managing the system temperature as high as possible without damaging the bacteria will have the most effective treatment.
2.3 Dissolved Oxygen
Micro-organisms are typically classified on their capability to utilize free oxygen molecules present in solution, which are referred to as aerobic bacteria. Anaerobic bacteria can only utilize oxygen present in organic and inorganic compounds such as carbohydrates, nitrates and sulphates. Some anaerobic species can shift their metabolism to utilize molecular dissolved oxygen to oxidize carbonaceous matter, and these are referred to as facultative bacteria.
Aerobic reaction breaks down complex carbonaceous compounds to carbon dioxide, nitrates, sulphates and extracellular matters, of which none of these elements produces an odour and explains why this has historically been the preferred treatment method. The rate-limiting factor is the availability of oxygen dissolved in water, which is very low. Treatment relies on mechanical addition of air through energy-intensive mixers or aerators, which contributes to high operational costs for these treatment facilities.
Anaerobic reaction relies on the reduction of compounds in solution to supply the oxygen for the bacterial metabolism, and as a result compounds such as sulphates and nitrates can be reduced to hydrogen sulphide and elemental nitrogen respectively. This is an attractive treatment solution where there are strict discharge limits regarding sulphates and nitrates. However, the generation of hydrogen sulphide can be a health and safety risk, as well as generating an odour in the surrounding area. The reaction rate for anaerobic breakdown is significantly slower than aerobic. So, this process is typically reserved for the sludge from aerobic treatment only, due to the lower volume to reduce reactor design requirements for the desired residence time. One of the by-products formed through anaerobic digestion is methane gas, which has a high energy content and can be harnessed for heat generation through combustion, or converted to electrical energy through a combustion generator.
2.4 pH
Different microbial groups have their tolerances for pH range, with bacterial growth optimized between 6.5 and 9.0. Carbon dioxide is a by-product of biological degradation, and therefore the presence of carbonates and bicarbonates are important as buffering reagents to prevent fluctuations in pH. It is especially important to monitor the pH during anaerobic digestion to ensure the pH remains stable and does not reach toxic levels, which can typically be addressed by adding limestone or hydrated lime to the solution as a buffering agent. Water with high total hardness occurring naturally will have a buffering effect on pH without the addition of chemicals required.
3 Aerobic Conditions
Due to aerobic micro-organisms receiving the most energy from the conversion of organic matter, this treatment process has faster reaction kinetics. It avoids the potential generation of odorous hydrogen sulfide formation. The reaction is explained by the simplified equation below:
Equation 2
The oxidation state of the compounds present in the reaction indicates the maximum quantity of energy available through the reaction. The more reduced state of the compound, the more energy it contains. Micro-organisms will attempt to utilize the compounds in such a manner to release the most amount of energy for the reproduction of the organism. The micro-organisms will attempt to oxidize the final product to the highest oxidation state, which is CO2 for the carbon components, and also why the aerobic micro-organisms have the highest growth rates.
Average raw wastewater pH is in the alkaline to neutral range, which means that the bulk of the ammonia is present as the ammonium ion (NH4+) and will be further oxidized to nitrates as per the following reaction:
Equation 3
Equation 3 is a simplification of the bacterial metabolism of two different species, Nitrosomonas and Nitrobacter, which complete the intermediate reaction from nitrite to nitrates (M. Von Sperling, 2007). It is important that the anoxic reaction also take place, which utilizes the hydrogen ions for the reduction of the nitrate to nitrogen gas. Natural waters with high alkalinity provide a buffer to prevent the pH from dropping too low, which could start adversely affecting the micro-organisms.
4 Anoxic Conditions
Once the oxygen has been depleted in solution, and there is a high concentration of nitrates present, an interim phase occurs, which is referred to as the anoxic zone. As indicated earlier, this phase is important to prevent the acidification of the solution by consuming hydrogen ions. Heterotrophic bacteria utilize nitrate as the electron donor instead of oxygen for their metabolic processes as seen below:
Equation 4
Nitrogen is formed as gas and could cause settling issues if it occurred within the clarifiers in the treatment process. So it is important to ensure there is sufficient time for the heterotrophic micro-organisms to ensure that nitrate concentration in the effluent meets the discharge standards.
5 Anaerobic Conditions
Anaerobic digestion is achieved by a balance between different species of bacteria which can be simplified as two groups, the acid formers and the methane formers (Design, pg 173). The conversion of organic matter to methane occurs in two stages, the acidogenic phase and the methanogenic phase. During the acidogenic phase, the organic matter is converted to organic acids such as acetate which does not effectively remove the organic matter from solution, but only changes the phase it is present. The methanogenic organisms utilize the organic acids to convert them to methane, carbon dioxide and water which converts the organic material to the gaseous phase and effectively achieves organic removal as per the equation below:
Equation 5
The acid formers produce organic acids which drop the pH and inhibits the growth of the methane-producing bacteria once the pH drops below 6.0. Further reduction of the pH, in the absence of a buffering element such as carbonates, will result in inhibition of the acid-generating bacteria below a pH of 4.0 and thereby render the treatment facility ineffective. If the feed stream has a high BOD5 requirement with low nutrients, the acid-forming bacteria predominantly utilize the nutrients and result in lowering of the pH. It is therefore recommended that the design caters for at least 50% of the nutrient requirements of an aerobic reactor to allow for sufficient nutrients available for the methane-producing bacteria to grow efficiently.
6 Dispersed and Attached Biomass Growth
Biological sewage treatment processes can be divided into two main classifications regarding the structural formation of the biomass, which is either dispersed or attached biomass growth. For dispersed growth, there is no supporting structure present in the facility for biomass to attach to, and it grows in the dispersed form, suspended in the liquid. Attached biomass growth processes actively provide a growth medium for the biomass to attach to and form a biofilm. Depending on the design of the system, the media can be permanently submerged or receive intermittent liquid discharges, and retains explicitly the media and accumulated biomass. The primary biological treatment principles are the same for either classification; however, the kinetics can differ significantly between the two processes.
6.1 Dispersed Growth
One of the main advantages to dispersed growth processes such as the activated sludge process is the agglomeration of cells to form a secondary structure referred to as floc. As the biomass growth continues in the floc structure, it becomes larger and gains mass. The advantage of this is that natural sedimentation occurs due to higher mass, causing the floc to settle out of solution, as well as entrainment of suspended organic matter that has not been sufficiently decomposed. It is important to take into account that the size of the floc which forms is predominantly determined by the rate of agitation and aeration taking place within the process and is an important operational parameter to monitor.
The formation of floc is dependent on operational efficiency. It can vary from 50 to 500 micron, which can result in significant concentration profiles for BOD and dissolved oxygen within the floc structure. On the boundaries of the floc, the bacteria can readily hydrolyze and metabolize the organic matter encountered in the medium. Moving towards the centre of the floc, there may be a nutrient deficiency which can result in a portion of the organisms reverting to anoxic metabolic processes, despite the presence of dissolved oxygen in solution.
The successful operation of an activated sludge treatment facility relies on a good floc formation throughout the process. This is achieved when there is sufficient aeration and mixing to create a homogeneous solution, with an adequate supply of oxygen throughout. However mixing and aeration should not be excessive to the point where it can cause shear forces stronger than the adhesive strength of the floc particles, which will cause breakage and reduce the treatment efficiency.
Another important consideration for dispersed biomass reactors is that the hydraulic retention time of the solution must be greater than the doubling time of the micro-organisms to give them sufficient time for cell growth. If the flow rate exceeds this design requirement, there is the risk of washing out the bacteria to the downstream processes and reducing the treatment efficiency.
6.2 Attached Growth
By providing an attachment media for micro-organisms inside the reaction vessel, it is possible to create much higher biomass concentrations in the process. Historically media such as gravel was the most widely applied, but development in attachment media to optimize available surface area has seen the application of rotating biodisc reactors as well as fixed bed reactors. The biomass grows through cell-to-cell interaction to form a densely populated structure along the surface of the attachment media. Nutrients are adsorbed from the solution at the outer layer of the biofilm and transported by diffusion through the layers of the micro-organisms to be metabolized. This creates a nutrient and oxygen concentration profile within the biofilm where the outer layer can have aerobic conditions, but once the oxygen is depleted there is a transition to anoxic conditions. Finally, fully anaerobic conditions are achieved at the innermost layers.
A distinct advantage of the attached growth principle is that the biomass is fixed within the reactor, and nutrients are absorbed from the liquid phase meaning that there is no addition of suspended solids to the influent water. This reduces the need for final clarification of the treated water, whereas dispersed media requires more efficient filtration that is typically achieved through rake clarifiers.
For the attached growth treatment processes, a homogeneous flow and nutrient distribution is a critical control parameter. If there is an overload of nutrients the biofilm can grow too thick and block flow in the trickling bed filter, or block the area between rotating biodisc treatment facilities. This will reduce the organic removal efficiency as well as cause an eventual collapse of the biofilm for the micro-organisms that are isolated from a feed source.
Insufficient nutrient feed to the biofilm will result in the inner anaerobic layer growing, which could create an imbalance between the by-products created causing poor growth conditions for the aerobic layers which can lead to collapse between the layers of the biofilm.
7 Maturation Ponds
Maturation ponds are widely utilized as a final effluent water quality polishing stage, whereby pathogens are removed to make the discharge water quality meet specific disinfection requirements. There is limited BOD removal achieved in maturation ponds, mostly related to sedimentation. Still, in a primarily aerated environment with maximum sunlight penetration, the primary focus is to remove faecal bacteria from a solution. The primary mechanisms affecting faecal bacterial removal are:
- Time and Temperature
- High pH >9
- High light intensity and dissolved oxygen concentration
Research done by Feachal et al. (1983) showed that increased time and temperature in ponds result in increased faecal bacteria die-off. Maturation ponds are designed to be shallow, which allow for adequate sunlight penetration which promotes algal growth. The algae increase in this environment and consume CO2 from solution at a faster rate than it can be produced by bacterial respiration. Carbonate and bicarbonate ions are dissociated to form hydroxyl ions and carbon dioxide, algae consume the CO2, resulting in a disproportionate rise in pH, which can exceed 10. Sunlight at specific wavelengths can damage faecal bacteria cells which further accelerate the die-off of cells and improving final effluent quality.
These factors are important to take into account when evaluating existing operational wastewater treatment facilities with maturation ponds as a final treatment step. Poor performance of the upstream processes can result in sedimentation within the maturation dams, reducing their residence time making treatment less efficient. Due to the high nutrients in these ponds, there is the risk of eutrophication taking place or reed beds establishing. While they have some polishing function, the sunlight penetration into the pond is severely compromised, and there is increased competition for the algal population. With proper maintenance, maturation ponds can be an effective effluent polishing step, and a much cheaper solution compared to active chemical dosing facilities.
8 Operational Monitoring Parameters for Wastewater Treatment Facilities
Because wastewater treatment facilities rely on the natural micro-organisms to achieve organic removal, these facilities run well with minimal operator inputs under normal design conditions. However, there are key parameters that need to be monitored to ensure optimal treatment conditions are being maintained for effective organic removal.
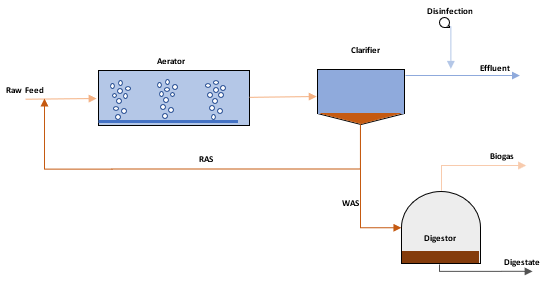
Figure 2: Wastewater treatment process diagram
8.1 Mixed Liquor Suspended Solids (MLSS)
To achieve maximum nutrient removal in an activated sludge process, sludge is recycled from the final clarifier and mixed with the feed to increase the micro-organism concentration within the reactor. This portion of sludge that is recycled is referred to as the Return Activated Sludge (RAS). In contrast, the remainder of the sludge is discharged either to digestion or to sludge drying beds which is referred to as the Waste Activated Sludge (WAS). Through the continuous recycle of sludge, the micro-organism concentration can be increased to 20 to 30 times of the natural concentrations, which achieves much more efficient nutrient removal.
The average target range for MLSS in an activated sludge process should be within the range of 2 000 to 4 000mg/L. The higher the MLSS value, the more effective the nutrient removal and shorter residence time is required, which allows for savings in the construction phase. There are practical limits which dictate an upper limit for MLSS values, such as maintaining the high dissolved oxygen required by the high concentration of micro-organisms and that the final stage clarifier has fixed settling capacity. Once biomass concentration exceeds the upper limit (depending on design), the final effluent quality will be compromised, and disinfection targets may not be achieved.
8.2 Dissolved Oxygen
Measuring dissolved oxygen can be described as one of the most critical parameters that need to be monitored and accurately controlled to ensure optimal performance. Injecting oxygen into the reactors is one of the most energy-intensive portions of any wastewater treatment works, and hence also a major contributor to treatment cost. It is therefore essential to supply sufficient oxygen to achieve nutrient removal, but not provide excessive oxygen as this will waste energy and can lead to other operational challenges.
It is recommended that dissolved oxygen concentration should be kept at 2mg/L in the aeration chamber, which ensures sufficient oxygen supply for the aerobic micro-organisms without using excessive energy. Suppose the oxygen is provided at high concentrations >4mg/L. In that case, it can cause conditions to favour the growth of filamentous bacteria which are much more difficult to settle and can cause operational challenges in the final clarifier process.
If there is insufficient dissolved oxygen, the nutrient removal process will be adversely affected, and a longer residence time will be required within the reactor. There will be a transition to anoxic conditions, which is required for some processes for effective nitrate removal. If complete anaerobic conditions are achieved, it can result in the generation of H2S and odour related complaints.
8.3 Hydraulic Retention Time
Wastewater treatment facilities are designed to allow for specific hydraulic retention time, dependent on the feed water BOD requirements. For low BOD requirement, the requirement can be 4 hours, up to 24 to 48 hours for high BOD industrial wastewater sources. Residential and industrial water flow rates leading to wastewater facilities have periodic fluctuations but should not typically exceed design rates under normal conditions.
In most developed countries, there are separate sewer systems for sewage and stormwater, so in the event of a storm, the wastewater treatment facility will not be impacted by such events. When the stormwater and wastewater collection in the same sewer systems, there is the risk of hydraulic overload during storm events. This can cause the flushing of micro-organisms from the treatment facility, which will result in no organic removal and the contamination of downstream facilities. This can be overcome with buffer dams for the raw water or potential effluent buffer systems such as maturation ponds which will cause additional polishing and improvement of the effluent water quality.
8.4 Solids Retention Time
The time micro-organisms spend within the reactor is an important control factor for these facilities, and is determined by the ratio of recycling of sludge to the wasting of sludge, and should be within the range of 2 to 8 days depending on the feed water BOD requirement.
Equation 6
The SRT is an indication of the amount of time that the mass of micro-organisms is circulated in the reactor, divided by the mass of micro-organisms in the waste stream, and the clarifier effluent. Typically the concentration in the clarifier effluent is disregarded as it is assumed close to zero if the clarifier is operating optimally. The mass of micro-organisms being sent to waste will either be for composting, drying for disposal to landfill or further processing through digestion and the possible recovery of biogas. If the digestion process is optimized, there is the possibility of energy recovery from the biogas generated, and the digestate which is rich in nutrients can be used for agricultural purposes.
8.5 Food to Mass Ratio
Food to mass ratio refers to the BOD of the raw feed water to the mass of micro-organisms in the reactor. The incoming BOD is equated to food for the micro-organisms, and if this is too high, some of it will pass through the reactor and contaminate the downstream processes. There is also the risk of viscous bulking, where the micro-organisms have too many nutrients as a feed source, and their physiological properties change to the extent where these micro-organisms do not settle well. When this ratio is too low, the population of micro-organisms change as these conditions favour the filamentous bacteria that are better at collecting the dispersed nutrients, which again result in poor settling in the final clarification process.
9 Emerging Wastewater Pollutants
Traditional wastewater treatment facilities have been designed to remove organic waste, but due to rapid industrialization, there are increasing cases where additional pollutants are present in solution. Some of these components are elemental metals, perfluoroalkyl substances (PFASs) and pharmaceutical and personal care products (PPCPs) which are still being investigated to understand their potential environmental impacts and possible health risks (Y. Zou, 2019).
PFASs have been produced since the early 1950s, due to their strong electronegativity and the small atomic size of fluorine which gives enhances properties to molecules such as increased acidity, higher surface activity at low concentrations, stability and water-repellency (Z.Wang et al., 2017). Due to their high stability, these synthetic compounds are accumulating in the environment, and their associated health risks are not sufficiently understood and require more research. The risks regarding pharmaceutical and personal care products (PPCPs) are related to their secondary impacts, as they cannot be metabolized by the humans or animals and also risk the building up in the natural environment.
A study done by Y. Zou et al. (2019) investigated the impact of these contaminants on algae and fish to determine their health and activity. Their findings indicate that metals have the highest risk to the health of fish and algae, whereas they found that the impacts on fish and algae differ significantly between PFASs and PPCPs. PPCPs were identified as having the next most significant impacts on algae and fish, whereas PFASs posed relatively low risks to these organisms.
Traditional wastewater treatment facilities rely on biological processes to affect organic removal, whereas these pollutants are inorganic and natural and not affected by these processes. It should be noted that under anaerobic conditions and the presence of sulphates and metals, the sulphate can be reduced to sulfide and result in the precipitation of metal sulfide compounds, which is one way that existing treatment facilities can be modified to achieve metal removal. Further work is required to better understand the impact of PFASs and PPCPs, as well as the processes required for their removal.
10 Wastewater Effluent Reuse Opportunities
There are specific regulations regarding the reuse of wastewater which depend on the reuse application, and due to the various treatment technologies implemented for wastewater treatment, they may limit the final effluent quality that can be achieved. Typical residential wastewater can be treated efficiently to remove all organic contaminants, and with effective disinfection, the final effluent may resemble potable water quality. For health and safety concerns, wherever wastewater effluent is reused for potable standards, the double barrier system is implemented. This is where reverse osmosis membranes and advanced chemical oxidation is used to ensure bacterial, inorganic compounds and elements are removed to ensure the health of the final consumers. These technologies are expensive due to advanced equipment, high energy and chemical reagent requirements.
One avenue for treated wastewater effluent reuse is in industrial process water streams where the industrial user has its wastewater treatment facilities. For industrial water where there are not very strict quality requirements, but the water is primarily used as a mode of transport and cannot affect the final product quality. Ineffective disinfection is the primary risk with reuse of effluent due to the risk of infecting the process water circuit where it is being used. Once micro-organism growth has established in industrial processes such as cooling towers or heat exchangers, it can be tough to remove. Due to the rapid growth of the micro-organisms, they can potentially form large colonies or biofilm, resulting in blocking of pipes and affecting equipment functionality. Ensuring that there is sufficient disinfection is critical for such applications, and there is online monitoring equipment capable of measuring free chlorine or oxygen reduction potential as an indicator.
The main consumer for treated effluent globally is for agricultural purposes, with different quality requirements depending on the type of crop that is grown or whether the water is used for irrigation of public areas. A review done by F. Shoushtarian & M. Negahban-Azar (2020) provides an extensive summary of the various guidelines and water quality limits that are used in different countries across the world. The different parameters and their limits are compared per country with the main differences highlighted to indicate how the standards change per area. BOD5 is used as a general performance indicator for wastewater treatment facilities, and some of the results are summarized in the table below as per the review:
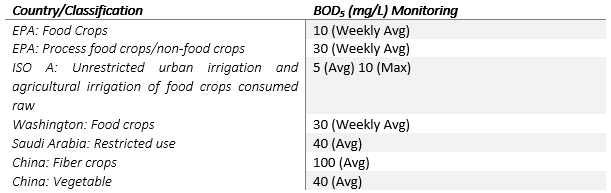
Table 1: Comparison of effluent reuse BOD5 concentration limits
BOD5 is, however, only one of the parameters that need to be monitored to determine whether the effluent is fit for use, but multiple other parameters need to meet the limits. Residual free chlorine is an important parameter to monitor as it is an indication of effective disinfection; however, if the values exceed 0.5mg/L there, it can affect some types of crop growth. Guidelines and limits will have to be updated on a global scale as more research is done into the new emerging pollutants and there are new results available on the potential health and safety impacts of these pollutants to humans, animals and different ecosystems. Wastewater treatment facilities will have to be optimized and expanded in the future as the contaminants in our water sources increase, and treatment requirements become more onerous. Continuous development in wastewater treatment technologies is of critical importance in our fast-paced globalizing economy.
11 References
E.A.R. Ouano, 1983, Principles of wastewater treatment, Biological Processes, Volume 1.
- Shoushtarian & M. Negahban-Azar, 2020. Worldwide regulations and guidelines for agricultural water reuse: a critical evaluation. Water Journal, 12, 971.
- Von Sperling, 2007, Basic Principles of wastewater treatment, Biological wastewater treatment series, Volume 2.
- Zou, J. Meng, M. Zhang, S. Chen, B. He, H. Zhao, Q. Li, S. Zhang & T. Wang, 2019. Which type of pollutants needs to be controlled with priority in wastewater treatment plants: traditional or emerging pollutants? Environment International, Volume 131.
- Wang, J.C. DeWitt, C.P. Higgins & I.T. Cousins, 2017. A never-ending story of per- and polyfluoroalkyl substances (PFASs). Environmental Science and Technology, Volume 51.